- 1College of Resources and Environment, Yunnan Agricultural University, Kunming, China
- 2The Germplasm Bank of Wild Species, Yunnan Key Laboratory for Fungal Diversity and Green Development, Kunming Institute of Botany, Chinese Academy of Sciences, Kunming, Yunnan, China
- 3Department of Crop and Forest Science, University of Lleida, Lleida, Spain
- 4Centre of Excellence for Soil Biology, College of Resources and Environment, and Chongqing Key Laboratory of Plant Resource Conservation and Germplasm Innovation, School of Life Sciences, Southwest University, Chongqing, China
- 5School of Biological Sciences, University of Western Australia, Perth, WA, Australia
- 6Guizhou Kangqunyuan Biotechnology Co., LTD, Liupanshui, Guizhou, China
Black truffles and white truffles are widely studied around the world, but their effects on plant growth and physiological responses, and on the mycorrhizosphere bacterial community of the host plant remain unclear. Here, mycorrhizal colonization of Castanopsis rockii by Tuber indicum (Chinese black truffle) and T. lijiangense (Chinese white truffle), respectively, was induced in a greenhouse study, and their effects on host growth, physiological responses and mycorrhizosphere bacterial communities were compared. The results show that colonization of both Tuber species significantly increased leaf photosynthetic rate, leaf P concentration and mycorrhizosphere acid phosphatase activity, as well as richness of mycorrhizosphere bacterial communities of C. rockii seedlings. However, T. indicum colonization on the one hand significantly decreased tartrate content, bacterial acid phosphatase, phoC gene abundance in the mycorrhizosphere, and peroxidase (POD) activity of ectomycorrhizal root tips, but on the other hand increased mycorrhizosphere pH and superoxide dismutase (SOD) of ectomycorrhizal root tips, compared to T. lijiangense colonization. Moreover, principal coordinate and β-diversity analyses show significant differences in mycorrhizosphere bacterial community composition between T. indicum and T. lijiangese colonized C. rockii seedlings. Finally, the relative abundance of the bacterium Agromyces cerinus significantly correlated to mycorrhizosphere acid phosphatase activity and leaf P concentration, suggesting that this bacterium might play an important role in P mobilization and acquisition. Overall, these results suggest that T. indicum and T. lijiangense differently regulate their host plant’s physiological responses and mycorrhizosphere bacterial community.
Introduction
Ectomycorrhizal (ECM) fungi form mutually beneficial associations with host plant roots, which are characterized by the presence of a fungal mantle that envelops lateral roots and a Hartig net that surrounds root epidermal and/or cortical cells (Smith and Read, 2008). About 6,000 woody plant species of mostly temperate and boreal forests form associations with ectomycorrhizal fungi (van der Heijden et al., 2015). A variety of factors have been found to affect the development of symbiosis, such as the host species, surrounding environment and management practices (Leonardi et al., 2013; Li et al., 2018). Ectomycorrhizae can promote plant growth, and provide resistance to stress and soil pathogens through an enhanced uptake of soil nutrients (Smith and Read, 2008; Cairney, 2011; Nehls and Plassard, 2018). Ectomycorrhizae can also release carbon- (C) and nitrogen-(N) containing exudates, including amino acids, organic acids and enzymes to mobilize less available nutrients from soil (Smith and Read, 2008; Cairney, 2011; Wang and Lambers, 2020; Wang et al., 2021), and alter root carbon exudation and rhizosphere bacterial communities (Wang et al., 2021). Furthermore, ECM fungi colonization can drive changes in rhizosphere bacterial communities thus affect the formation and eco-physiological roles of ectomycorrhizae (Berendsen et al., 2012), as well as host plant performance (Izumi et al., 2006; Bulgarelli et al., 2013).
A group of fungi belonging to the genus Tuber (Ascomycota, Pezizales), which produce edible hypogeous ascocarps called truffles (Benucci and Bonito, 2016), are typical ectomycorrhizal fungi that form symbiotic relationships with several genera of trees, such as Pinus, Quercus and Castanopsis (Trappe et al., 2009; Benucci et al., 2012; Marozzi et al., 2017; Freiberg et al., 2021; Huang et al., 2021). The ECM symbiosis spans most of the life cycle of truffles, and the quality of truffle-colonized seedlings early in the symbiosis development is an important factor that determines the production of truffle fruiting bodies in plantations (Andres-Alpuente et al., 2014; Mello and Balestrini, 2018). The genus Tuber displays a widely geographic distribution, from North Europe, North Africa, Asia, to North and South America (Bonito et al., 2013; Lancellotti et al., 2016; Le Tacon, 2016). Some Tuber species such as T. melanosporum and T. magnatum have highly economic value because of their specific taste and unique fragrance (Bertini et al., 2006; Mello et al., 2006). Tuber-associated host tree species including Pinus, Quercus and Populus have important ecological values as major reforestation species (Liu et al., 2014). Studies have shown that ectomycorrhizae formed by Tuber species promote the growth of their host plants (Alvarez-Lafuente et al., 2018; Wang et al., 2021; Huang et al., 2022). Tuber ectomycorrhizae can also alter root carbon exudation and metabolic profiles of host plants, as well as mycorrhizosphere microbial communities (Benucci et al., 2016; Deveau et al., 2016; Li et al., 2018; Li et al., 2019; Yang et al., 2019; Marjanović et al., 2020; Wang et al., 2020; Wang et al., 2021; Marozzi et al., 2022).
Tuber indicum, known as Chinese black truffle, has been successfully cultivated with Quercus aliena in southwest China (Wang et al., 2020). T. indicum has similar morphological characteristics and a close phylogenetic relationship with T. melanosporum (Murat et al., 2008) and associates with more than 20 tree species belonging to different plant families such as Fagaceae, Juglandaceae, Pinaceae and Salicaceae (Huang et al., 2020; Wang et al., 2020). T. lijiangense, known as Chinese white truffle, has been firstly described from a natural Pinus yunnanensis forest in Yunnan, southwest China, and its ascomata have a strong pleasant aroma (Fan et al., 2011). Both species are expensive in local markets in southwest China (Liu et al., 2011; Wan et al., 2016). Studies have shown that T. indicum could increase soil organic matter in rhizosphere of Q. aliena, affect host uptake of phosphorus (P) and N, and alter rhizosphere microbial communities (Li et al., 2018; Zhang et al., 2020). However, little information is available about effects of T. lijiangense colonization on the performance of host plants and soil characteristics. Therefore, whether the colonization by T. indicum or T. lijiangense could have similar or different impacts on host plant growth, physiological responses and rhizosphere microbial community are still largely unknown.
Castanopsis species belonging to Fagaceae family are usually large canopy trees, widespread generalists growing in different habitats with varying altitudes and soil types (Dong et al., 2009; Cheuk and Fischer, 2021). Some species in the Castanopsis genus are comparatively fast-growing, suitable for controlling soil erosion and reforestation (Ren et al., 2008; Dong et al., 2009; Cheuk and Fischer, 2021). For instance, Castanopsis rockii, an endemic woody species and a potential Tuber host, is widely utilized as a timber tree, in Yunnan, China. The objectives of the study were to assess whether: 1) both Tuber species could form ectomycorrhizae with C. rockii under controlled conditions; 2) T. indicum and T. lijiangense alter plant growth, nutrient uptake and physiological responses in C. rockii mycorrhizosphere; and 3) T. indicum and T. lijiangense mycorrhization of C. rockii may shape the bacterial community structure and diversity in the mycorrhizosphere. The expected results will provide valuable information in improving field cultivation of these two ecologically and economically important Tuber species.
Materials and methods
Seedling cultivation and ectomycorrhizal inoculation
Seeds of C. rockii were collected from the Kunming Botanical Garden (KIB). Before germination, seeds were soaked in tap water for one day, with an initial water temperature of 55°C (Mao et al., 2013), then surface sterilized in sodium hypochlorite (2% available chlorine) for 2 h. After being thoroughly rinsed in distilled water, seeds were germinated in a large plastic crate, which was lined with a cotton mesh that held a sterilized growth substrate (perlite: vermiculite: water at 1:1:1), in a greenhouse at KIB, under natural conditions.
Fresh ascomata of T. indicum and T. lijiangense were purchased from local markets in Kunming, Yunnan, China. After being identified by both morphological and molecular techniques, these ascomata were then sliced and dried at room temperature for over 72 h and stored in plastic boxes at 4°C until use. The synthesis of ECM seedlings was performed according to our previous study (Huang et al., 2022). In brief, 3-month-old seedlings of similar size were selected, washed and transplanted into sterilized substrate (peat: vermiculite: perlite: water at 2:3:1:1) at pH 7.3, adjusted with calcium carbonate (0.19 g/L) and magnesium carbonate (0.1 g/L). The inocula of T. indicum and T. lijiangense were obtained by blending the ascomata, which had been soaked in non-sterile distilled water for 24 h at 4°C. Each seedling was inoculated with 10 mL spore slurry (~5 ×106 spores) in May 2020. Meanwhile, each uninoculated control seedling received 10 mL of sterilized spore slurry. Seedlings were grown in 688-ml square plastic pots (13.2 × 6.4 × 9.1 cm), with one seedling per pot. A total of 38 C. rockii seedlings were grown in a random arrangement of pots in a greenhouse at KIB. Of them, 16 were inoculated with T. indicum, 16 with T. lijiangense, and 6 were the control group. Seedlings were watered two or three times per week with tap water. No fertilizer was added during the whole experiment.
Ectomycorrhizal analyses
The macro-morphological characters of T. indicum and T. lijiangense mycorrhizae associated with the seedlings were examined and photographed 6 months after inoculation under a stereomicroscope (Leica S8AP0, Leica Microsytems, Wetzlar, Germany). To confirm fungal identities, 5 colonized root tips from each seedling were collected for DNA extraction. The Internal Transcribed Spacer (ITS) region of the ribosomal DNA was amplified with the ITS1F/ITS4 primer pair (White et al., 1990; Gardes and Bruns, 1993). PCR analyses were carried out on a LifeECO thermocycler (LifeBioer Technology, China) in a final volume of 25 μL containing 1μL DNA template, 1 μL of each primer (5 μM), 12.5μL of 2×Taq Mastermix, 9.5 μL ddH2O. The amplifications were performed with the following cycling parameters: 94°C for 5 min, followed by 35 cycles at 94°C for 1 min, 50°C for 1 min, 72°C for 1 min, and with a final extension at 72°C for 10 min. Three microliters of each PCR product were run on 1% (w/v) agarose gels and visualized by staining with ethidium bromide in a Molecular Imager Gel Doc EX system (Syngene, Shanghai, China). PCR products were Sanger sequenced in one direction by TsingKe Biological Technology, Kunming, China, and queried against published sequences deposited in GenBank database.
The mycorrhizal colonization rates were determined by following the method and criteria described by Murat (2015). Briefly, the density of mycorrhizal colonization throughout the whole root system was visually estimated and scored. All the mycorrhized (T. indicum: n = 12, T. lijiangense: n = 3) and control (n = 6) seedlings were used for measuring their plant growth and physiological parameters. The mycorrhizosphere substrate was used for the determination of substrate properties (T. indicum: n = 10, T. lijiangense: n = 3, control: n = 5), bacterial community (T. indicum: n = 5, T. lijiangense: n = 3, control: n = 5), and phoC and phoD gene abundance (T. indicum: n = 4, T. lijiangense: n = 3, control: n = 4).
Measurement of plant growth and plant physiological parameters
A digital caliper was used to measure the following plant growth parameters: (1) plant height (from root collar to top of stem), (2) stem diameter (about 2 cm from the potting mix surface) and (3) canopy diameter (the maximal distance between the apices of opposite leaves). Numbers of leaves were simultaneously recorded.
The investigated physiological parameters of C. rockii seedlings included leaf photosynthetic parameters, major leaf nutrients, root superoxide dismutases (SOD) activity, and root peroxidase (POD) activity. Using a portable gas exchange fluorescence system GFS-3000 (Heinz Walz GmbH, Effeeltrich, Germany), photosynthetic rate, transpiration rate, stomatal conductance and intercellular CO2 concentration in the 2nd fully expanded top leaf were measured between 9:30 am and 11:30 am, under 1000 μmol active radiation m−2 s−1, 400 ppm CO2, 25°C, and 50% relative humidity. Healthy and mature leaves in the middle of the seedling canopy were harvested to determine their fresh and dry (75°C for 48 h) biomass. The dried leaves were grounded to fine powder using a high-flux tissue mill (Scientz-48, Schneider Electric (China), Beijing, China). Leaf N was determined with a Vario MAX CN (Elementar, Langenselbold, Germany). Leaf total P, potassium (K), calcium (Ca) and manganese (Mn) were determined with an Inductively Coupled Plasma Atomic Emission Spectrometer (Thermo Jarrell Ash, MA, USA). Activity of root SOD and POD was determined according to the nitro-blue tetrazolium (NBT) (Shafi et al., 2015) and guaiacol method (Rácz et al., 2018), respectively.
Determination of mycorrhizosphere pH, phosphatase activity and exudates
The mycorrhizosphere substrate was analyzed to determine mycorrhizosphere pH and phosphatase activity. About 4 g of fresh mycorrhizosphere substrate from each plant were carefully sampled using tweezers and spatulas, then divided into two parts: the first was stored at -20°C for DNA extraction and the second was air-dried at room temperature for pH and phosphatase activity measurements. The mycorrhizosphere pH was measured at a 1:5 ratio of substrate and water (W: V). The activities of rhizosphere acid or alkaline phosphatase (S-ACP or S-ALP) were measured separately using their respective kits (Solar-bio®, Beijing, China), following the manufacturer’s instructions.
After the sampling of mycorrhizosphere substrate, the entire root system with the remaining mycorrhizosphere substrate was transferred into a flask containing 150 mL 0.2 mM CaCl2 and 0.01 g L-1 Micropur (Katadyn Products, Kemptthal, Switzerland) solution to ensure cell integrity and inhibit the activity of microorganisms. Roots were gently dunked for 150 s to get rhizosphere extracts (Wang et al., 2021), which were then centrifuged (1000 rpm, 10 min), and 10 mL of the supernatant was freeze-dried for 3 days, the dried residue was re-suspended in 2 mL deionized water, centrifuged (1000 rpm, 10 min) again and filtered through a 0.45 μm membrane filter. Total organic carbon (TOC) was measured by a Nano-300 micro-spetrophotometer (ALLSHENG, Hangzhou, China) at 254 nm, with an injection volume of 1 μL (Deflandre and Gagné, 2001). The TOC concentrations were determined according to their standard curves. Organic anions were determined through a HPLC (Agilent Technologies, Tokyo, Japan) process. A UV detector (SPD-20A) monitored at 210 nm was used for the analysis of the organic anions: the injection volume was 10 μL and sample components were separated using a ZORBAX SB-Aq (4.6 × 250 mm, 5 μm) StableBond analytical column (Agilent, Delaware, USA) at 35°C column oven temperature and 10 min running time. The mobile phase was 2% acetonitrile with 0.1% H3PO4, at a flow rate of 1.0 mL min-1. The organic anions were identified by comparing their retention times with standards and their concentrations were determined according to their standard curves (Wang et al., 2021).
qPCR quantification of phoC and phoD genes
Genomic DNA in 0.2 g of mycorrhizosphere substrate was extracted using a DNeasy® PowerSoil® Kit (QIAGEN GmbH, Hilden, Germany) following the manufacturer’s instructions. Extracted DNA was stored at -20°C until use. Substrate water content was calculated after oven-dried at 60 °C for 48 hours. The bacterial phoC and phoD genes were amplified with primers phoC-F (5’-CGGCTCCTATCCGTCCGG-3’), phoC-R (5’-CAACATCGCTTTGCCAGTG-3’) and phoD-F (5’-CAGTGGGACGACCACGAGGT-3’), phoD-R (5’-GAGGCCGATCGGCAT GTCG-3’), respectively (Fraser et al., 2017), on an ABI 7500 Real-Time PCR System (Thermo Fisher Scientific, Inc., MA, USA). All qPCRs were run in duplicated 10 μL reactions comprising 5 μL of 2x SYBR Green PCR Mix (BioRoYee, Beijing, China), 0.4 μL of each primer (0.4 μM), 1 μL of 1:20 diluted metagenomic DNA, and nuclease free sterile water. The two-step protocol for phoC was as follows: 3 min at 95°C, followed by 40 cycles of 10 s melt at 95°C and 30 s annealing and elongation at 58°C (Fraser et al., 2017). The three-step cycling conditions for phoD were as follows: 1 cycle at 94°C for 4 min, 40 cycles of 94°C for 45 s, 57°C for 30 s, 72°C for 45 s (Fraser et al., 2015). A melting step followed the amplification to ensure specificity of the reaction, from 60°C to 95°C ramping 0.1°C every second. Standard curves were prepared by 10-fold serial dilution of cloned plasmids. The copies of phoC and phoD gene were determined as per gram dry substrate based on standard curves.
Mycorrhizosphere bacterial DNA extraction and 16S rRNA sequencing
Bacterial DNA was extracted from 0.2 g of fresh mycorrhizosphere substrate using Powersoil™ DNA isolation kits (MoBio, San Diego, CA, USA) according to the manufacturer’s instructions. The V3-V4 region of the bacterial 16S rRNA gene was amplified using the forward primer 338F (5′-ACTCCTACGGGAGGCAGCA-3′) and the reverse primer 806R (5′-GGACTACHVGGGTWTCTAAT-3′). Purified amplicons were pooled, and pair-end sequenced on an Illumina MiSeq Platform, Miseq-PE250 (Personalbio®, Shanghai, China). The original paired-end sequencing data were saved as the FASTQ format. The raw reads were analyzed using a QIIME2 (2019.4) software to trim off adaptors, barcodes, primers and low-quality reads. The obtained sequences with ≥ 97% similarity were merged as an operational taxonomic unit (OTU) (Edgar, 2010). The Bray-Curtis distance-based dissimilarity distance, Observed species, Simpson, Shannon and Chao1 diversity index, principal coordinate analysis (PCoA) and a Venn diagram with shared and unique OTUs were performed on a Genescloud platform of Personalbio® to evaluate the bacterial community differences among non-ectomycorrhizal control, T. indicum and T. lijiangense ectomycorrhizal samples. Raw sequence data have been deposited in the NCBI Sequence Read Archive database under the bioproject identifier PRJNA854794.
Statistical analysis
R software (version 3.2.3) was used for statistical analyses. Because the sample sizes are not equal across different treatments, we used unbalanced analysis of variance (ANOVA) to examine mycorrhizal inoculation effects on plant growth parameters, photosynthetic parameters, leaf nutrient concentrations, leaf water content, root POD and SOD activity, mycorrhizosphere pH, phosphatase activity, mycorrhizosphere exudates, phoC and phoD gene abundance. Data were checked for normality before analysis. Significant mycorrhizal inoculation effects were examined in more detail using Tukey honestly significant difference tests, to determine differences among T. indicum colonization, T. lijiangense colonization and un-inoculated control group. Spearman correlation analyses with Bonferroni correction were used to examine the correlations between relative abundances of the 20 most abundant OTUs and measured plant or substrate parameters including plant growth parameters such as photosynthetic parameters, leaf nutrient concentrations, leaf water content, root POD and SOD activity, mycorrhizosphere pH, phosphatase activity and mycorrhizosphere exudates. The Spearman correlation analysis was performed using genescloud tools, a free online platform for data analysis (https://www.genescloud.cn). The PCoA analysis was based on a Bray-Curtis distance at the OTU level. For all statistical analyses, a significance level of P < 0.05 was used.
Results
Ectomycorrhizae formation
Six months after inoculation, twelve out of sixteen seedlings (75%) showed T. indicum colonization, while only three out of sixteen seedlings (19%) showed T. lijiangense mycorrhiza. The ectomycorrhization was established with 50-70% colonization rates in all mycorrhizal seedlings by a rough estimation. Ectomycorrhizal root systems formed by T. indicum and T. lijiangense were simple or monopodial with lateral tips, mostly ramified in a monopodial-pinnate pattern. The mantle surface of T. indicum mycorrhizae was smooth to hairy or slightly woolly, with some woolly emanating hyphae, while the mantle surface of T. lijiangense mycorrhizae often had abundant spiky emanating hyphae (Figure 1). ITS sequences from T. indicum and T. lijiangense mycorrhizae formed with C. rockii had an identity of 99.66% and 99.83%, respectively, with published sequences of T. indicum (GU979058) and T. lijiangense (KP276188) from GenBank. The sequences of T. lijiangense (NO920998) and T. indicum (NO920999) ectomycorrhizae obtained in this study with C. rockii have been deposited in the GenBank. No ectomycorrhizae were found on the roots of control seedlings, and no other ectomycorrhizal fungi were detected in any of the tested seedlings.
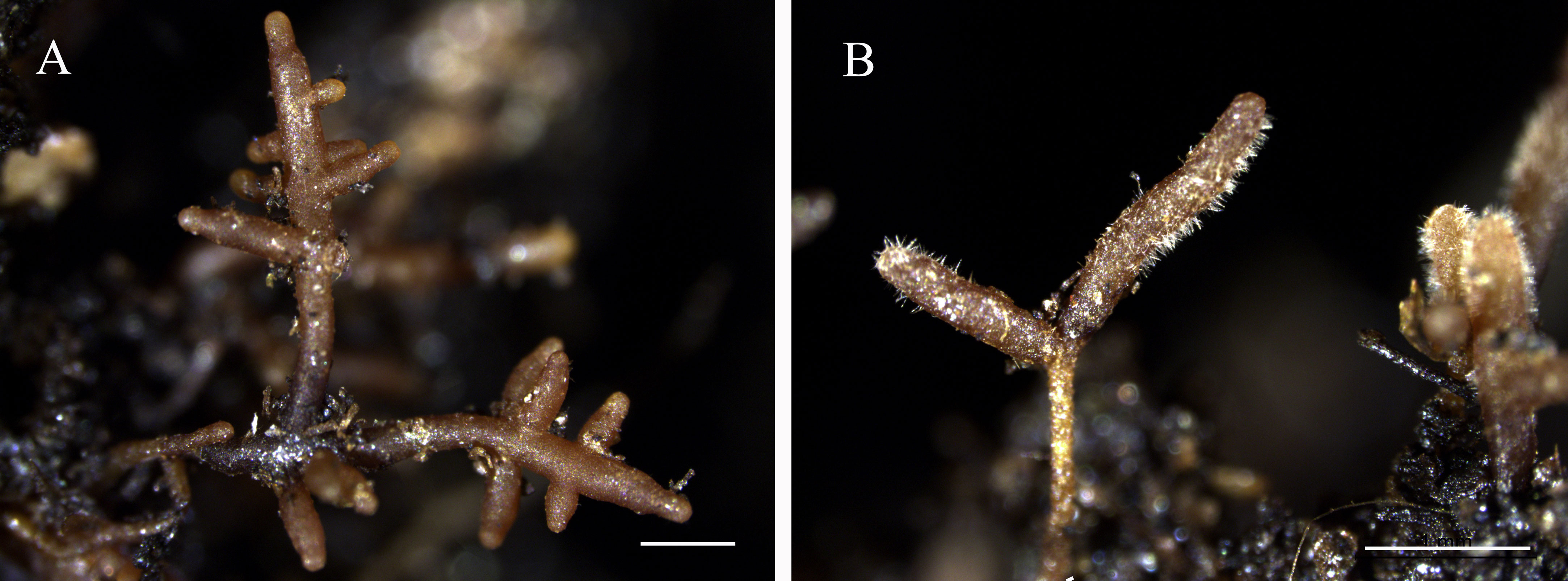
Figure 1 Ectomycorrhizae of Tuber indicum (A) and T. lijiangense (B) associated with Castanopsis rockii. (Bars = 1 mm).
Effects of mycorrhization on plant growth and physiological responses
Compared with uninoculated control seedlings, ECM colonization exhibited no significant effects on morphology and plant growth (Figures 2A–D). Leaf photosynthetic rate (F=7.21, P<0.01) (Figure 3C) and P concentration (F=5.18, P<0.05) (Figure 4B) were significantly improved by both fungi. However, transpiration rate (Figure 3A), leaf stomatal conductance (Figure 3B), intercellular CO2 concentration (Figure 3D), leaf concentrations of N (Figure 4A), K, Ca and Mn (Figures 4C–E) were not affected. Furthermore, T. indicum colonization significantly enhanced leaf water content (F=13.48, P<0.01), while T. lijiangense did not (Figure 4F). T. indicum and T. lijiangense colonization had opposite effects on root SOD and POD activity: T. indicum colonization markedly enhanced root SOD activity (F=4.97, P<0.05), while T. lijiangense colonization significantly reduced root POD activity (F=7.40, P<0.05) (Figures 5A, B). Compared to control treatment, T. indicum colonization significantly enhanced root SOD activity (F=8.54, P<0.05), but reduced root POD activity, while T. lijiangense had no significant impact on root SOD and POD activity (Figures 5A, B).
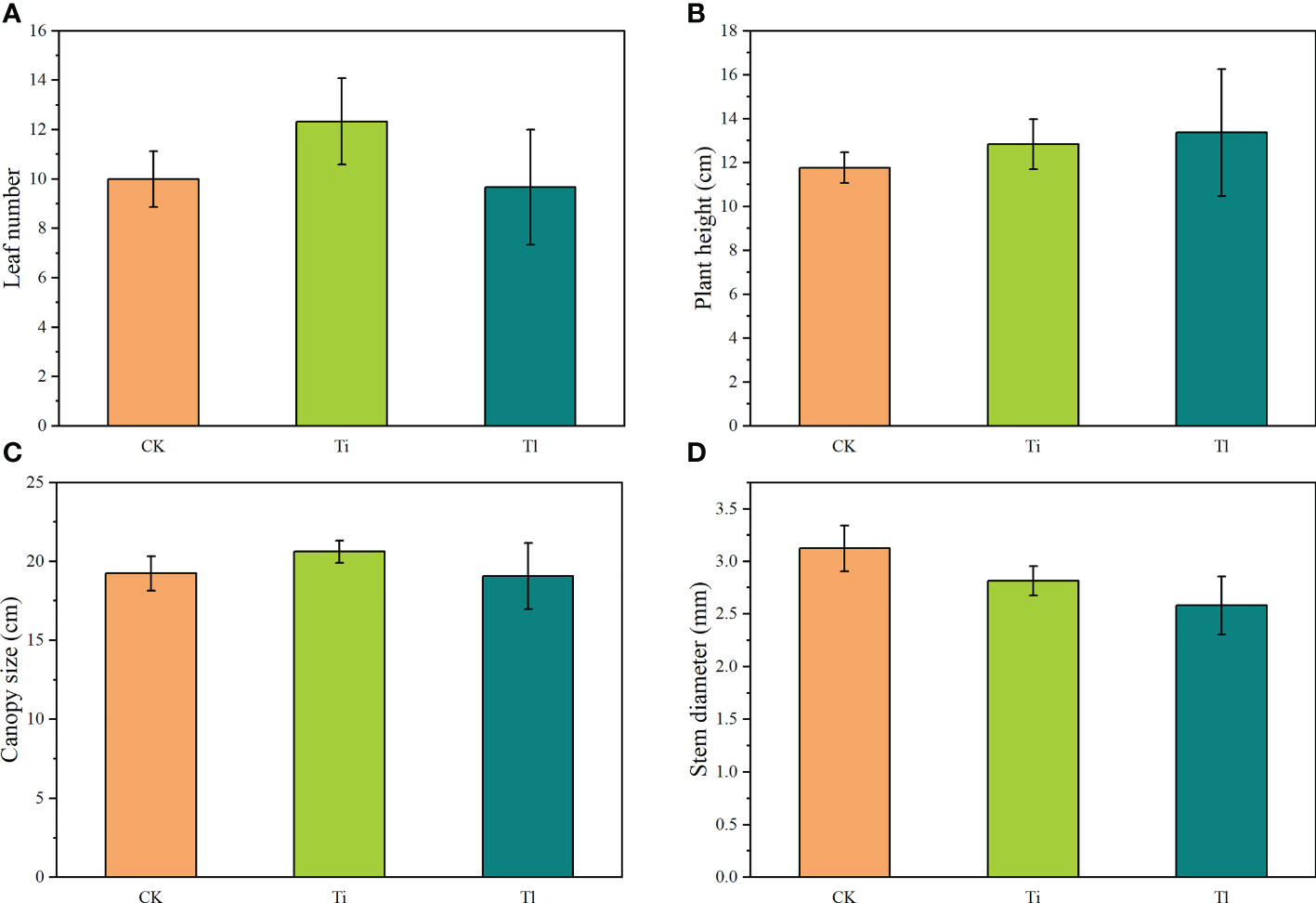
Figure 2 (A–D). Impact of colonization by Tuber indicum (Ti) (n=12) and T. lijiangense (Tl) (n=3) on Castanopsis rockii seedlings regarding plant growth parameters: leaf number (A), plant height (B), canopy size (C), and plant stem diameter (D). CK = control group (n = 6). Bars show means ± SE.
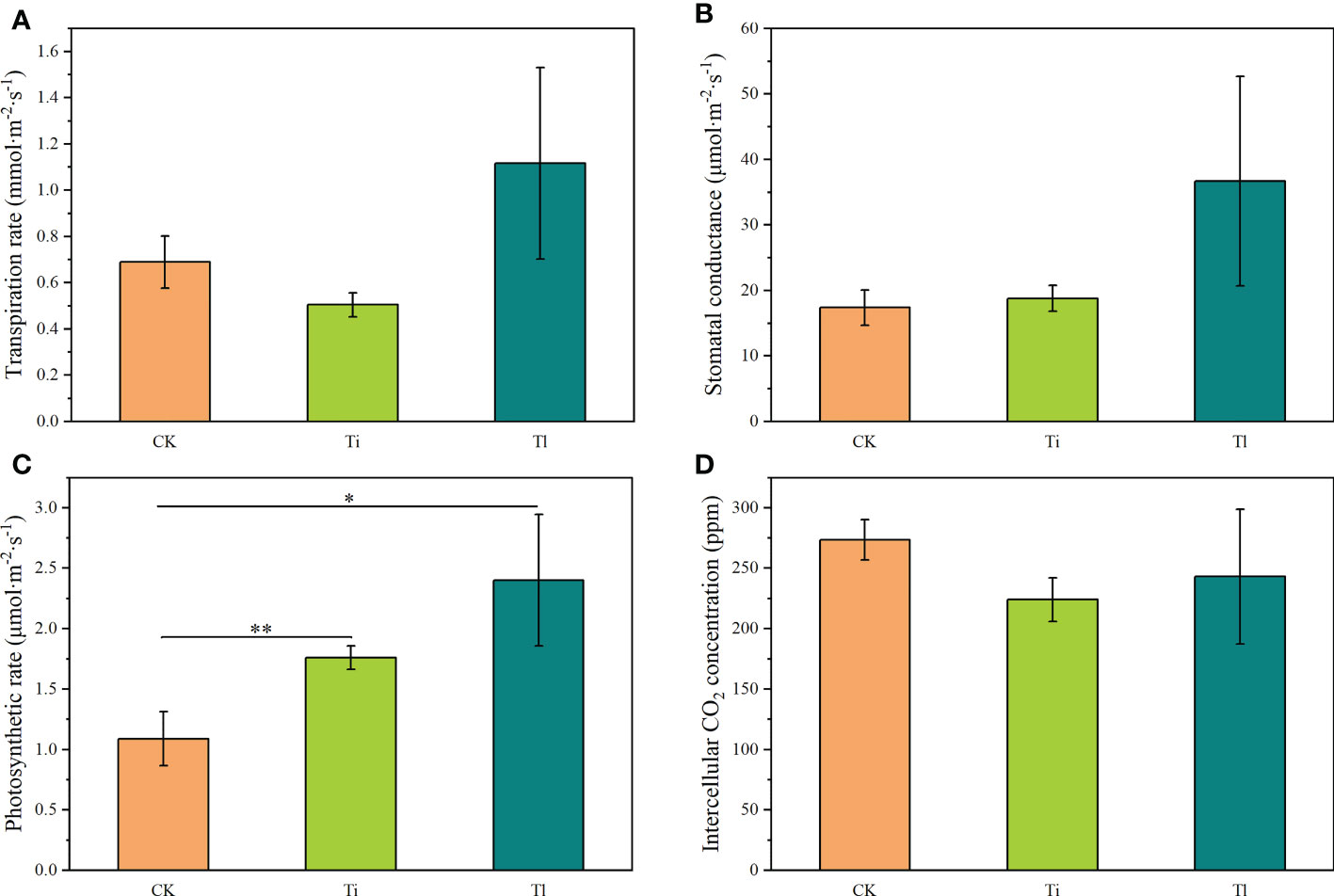
Figure 3 (A–D). Impact of colonization by Tuber indicum (Ti) (n =12) and T. lijiangense (Tl) (n= 3) on Castanopsis rockii seedlings regarding leaf photosynthetic parameters: transpiration rate (A), stomatal conductance (B), photosynthetic rate (C), intercellular CO2 concentration (D). CK = control group (n = 6). Bars show means ± SE. Significance at P<0.05 *, and P<0.01 **.
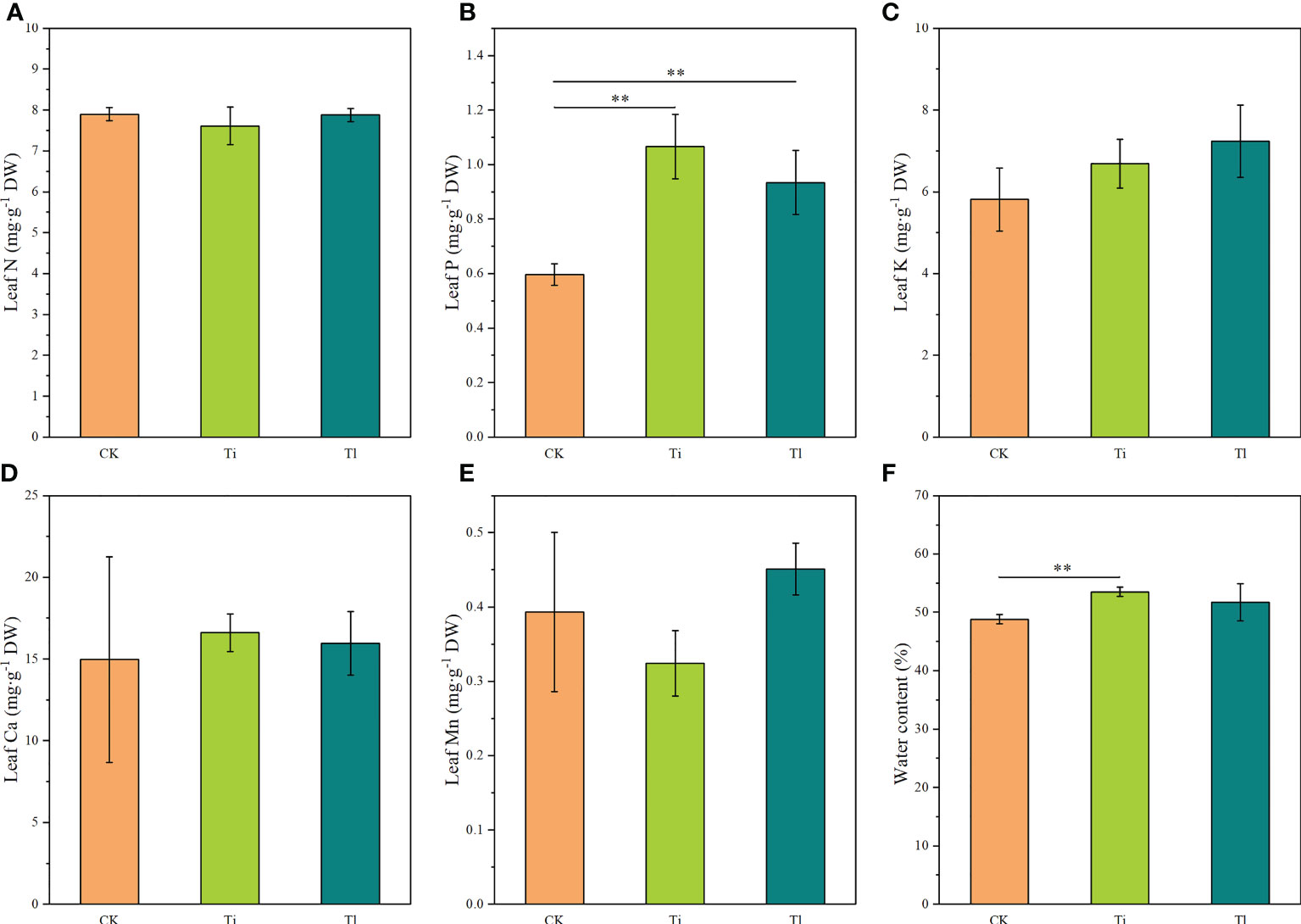
Figure 4 (A–F). Impact of colonization by Tuber indicum (Ti) (n =11) and T. lijiangense (Tl) (n = 3) on Castanopsis rockii seedlings regarding plant nutrient uptake of leaf nitrogen (A), leaf phosphorus (B), leaf potassium (C), leaf calcium (D), leaf manganese (E), leaf water content (F). CK = control group (n = 5). Bars show means ± SE. Significance at P<0.01 **.
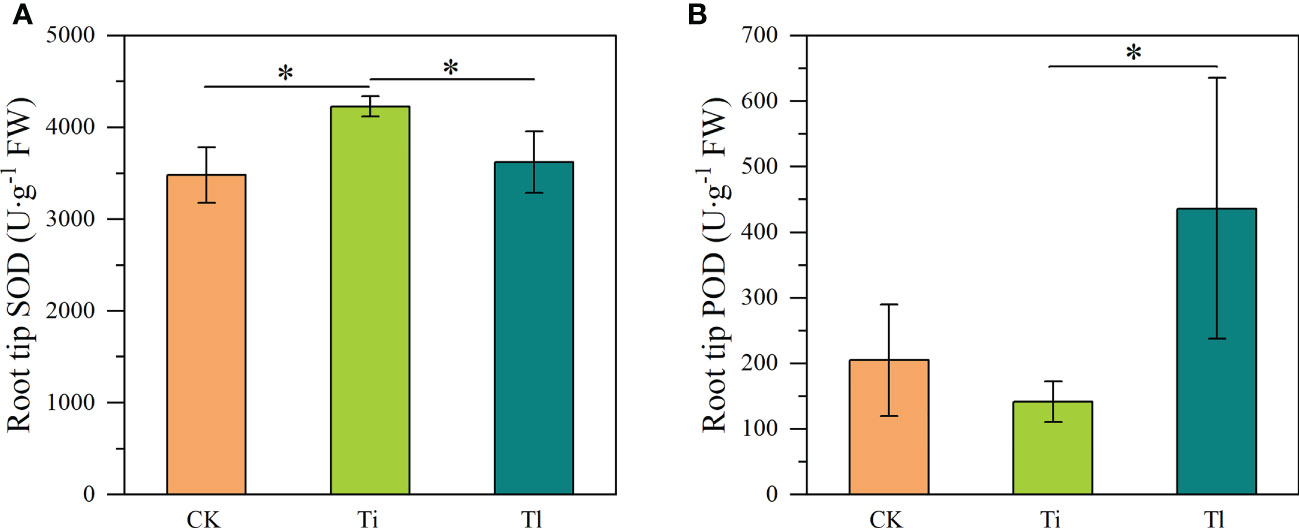
Figure 5 (A, B). Impact of colonization by Tuber indicum (Ti) (n =10) and T. lijiangense (Tl) (n = 3) on Castanopsis rockii seedlings regarding root oxidase activity of superoxide dismutase SOD (A) and peroxidase POD (B). CK = control group (n = 5). Bars show means ± SE. Significance at P<0.05 *.
Effects of mycorrhization on mycorrhizosphere pH, phosphatase activity and exudates
Seedlings associated with T. lijiangense had significantly lower mycorrhizosphere pH than control seedlings (F=17.71, P<0.01) and T. indicum colonized seedlings (F=5.10, P<0.05) (Figure 6A). In comparison with control group, T. indicum colonization significantly reduced tartrate accumulation (F=6.82, P<0.05) (Figure 6D). On the other hand, mycorrhizal colonization with both Tuber species did not significantly reduce mycorrhizosphere TOC (Figure 6B), and oxalate content (Figure 6C).
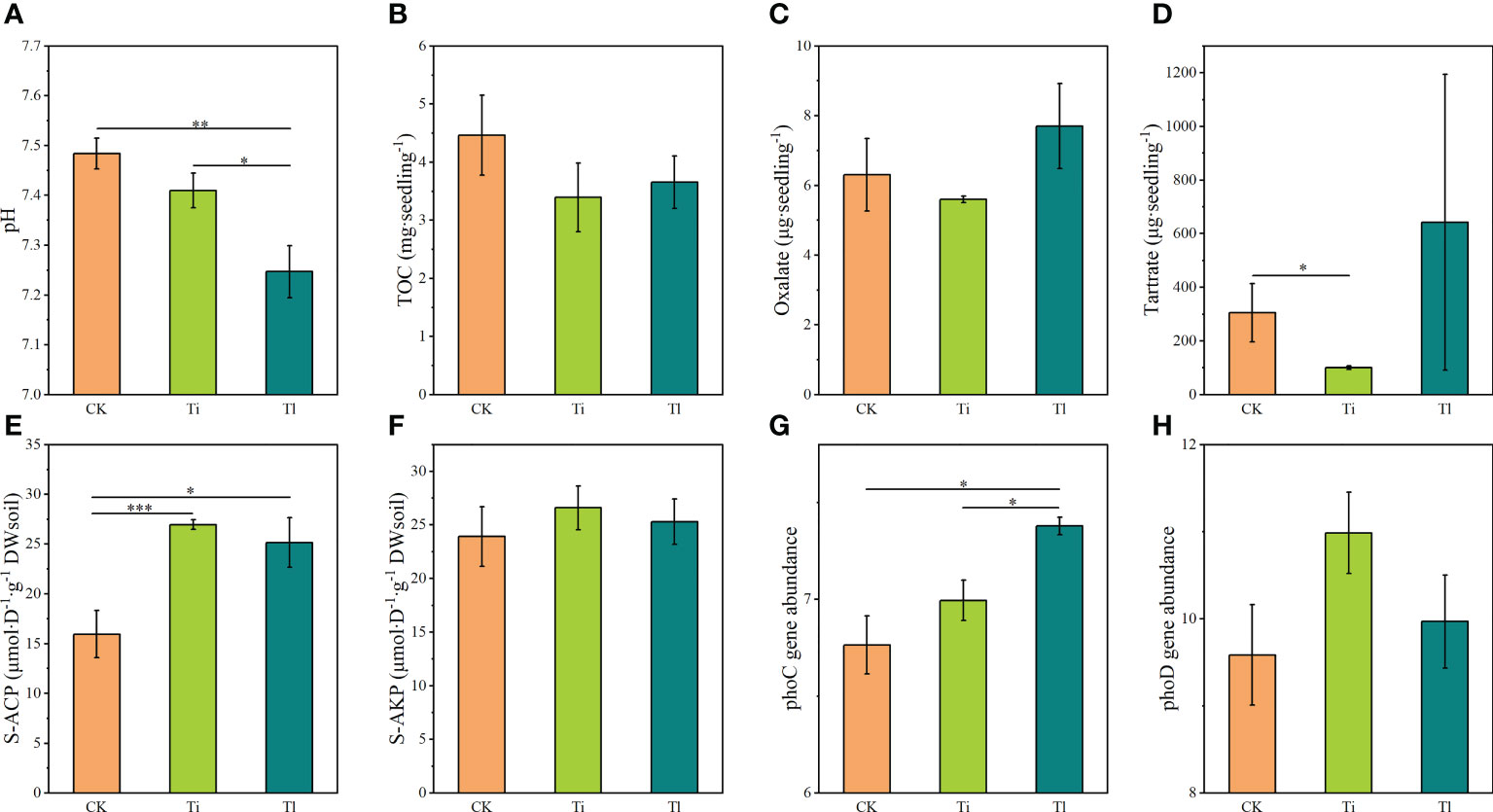
Figure 6 (A–H). Impact of colonization by Tuber indicum (Ti) and T. lijiangense (Tl) on Castanopsis rockii seedlings regarding mycorrhizosphere characterstics: pH (A), total organic carbon (B), oxalate (C), tartrate (D), acid phosphatase activity (E), alkaline phosphatase activity (F). For these parameters the sample size was as follows: Ti =10, Tl = 3, whereas for phoC gene abundance (G), and phoD gene abundance (H) it was Ti = 4, Tl = 3. CK = control group (n = 4). Bars show means ± SE. Significance at P<0.05 *, P<0.01 ** and P<0.001 ***.
Ectomycorrhizal colonization significantly improved mycorrhizosphere S-ACP activity of seedlings mycorrhized with both T. indicum (F=18.43, P<0.001) and T. lijiangense (F=6.41, P<0.05) in comparison with control group (Figure 6E), whereas S-ALP activity did not change (Figures 6F). T. lijiangense colonization significantly increased bacterial phoC gene abundance in comparison with T. indicum colonization (F=8.79, P<0.05) and the control (F=11.64, P<0.05), while T. indicum did not have any significant impact on this parameter (Figure 6G). The abundance of phoD gene was not affected by the colonization of both Tuber species (Figure 6H).
Effects of mycorrhization on mycorrhizosphere bacterial communities
Between 76,889 and 101,639 bacterial 16S rRNA gene reads were obtained from Illumina Paired-end sequencing for each sample after quality control procedures generated a total of 18,112 bacterial operational taxonomic units (OTUs) for further analysis. These bacterial OTUs were clustered into 35 phyla, 101 classes, 255 orders, 443 families, 855 genera and 1961 species. At the phylum level, Proteobacteria, Chloroflexi and Acidobacteria were the dominant bacterial phylum, with relative abundances of 47.9%, 15.9% and 14.9%, respectively. The relative abundance of Proteobacteria was 50.1%, 46.9% and 45.9% in the sample of plants colonized by T. indicum, T. lijiangense and control group, respectively.
The analyses of bacterial community indicated that T. lijiangense colonization showed significant differences regarding Observed OTU richness, Chao 1, Shannon and Simpson index (α-diversity, Figure 7A) compared with the control group, whereas T. indicum colonization showed significant differences only in Observed OTU richness and Chao 1 index. No significant differences were found between T. lijiangense and T. indicum colonization regarding all the α-diversity index (Figure 7A). Furthermore, bacterial communities in the mycorrhizosphere of C. rockii plants colonized by T. indicum and T. lijiangense revealed significant differences between them and in comparison with the control group regarding β-diversity (dissimilarity distance, Figure 7B total of 18,112 OTUs were obtained, with only 8% of them being shared among T. indicum, T. lijiangense and the control group (Figure 7C). In addition, T. indicum and T. lijiangense mycorrhizal samples and control group samples were clearly separated by PCoA (Figure 7D) Mycorrhizosphere communities of plants inoculated with T. indicum showed a significantly higher relative abundance of Firmicutes than control plants (F=14.34, P<0.01), and showed significantly less relative abundance of Chloroflexi than colonization by T. lijiangense (F=14.26, P<0.01) (Figure 8A).
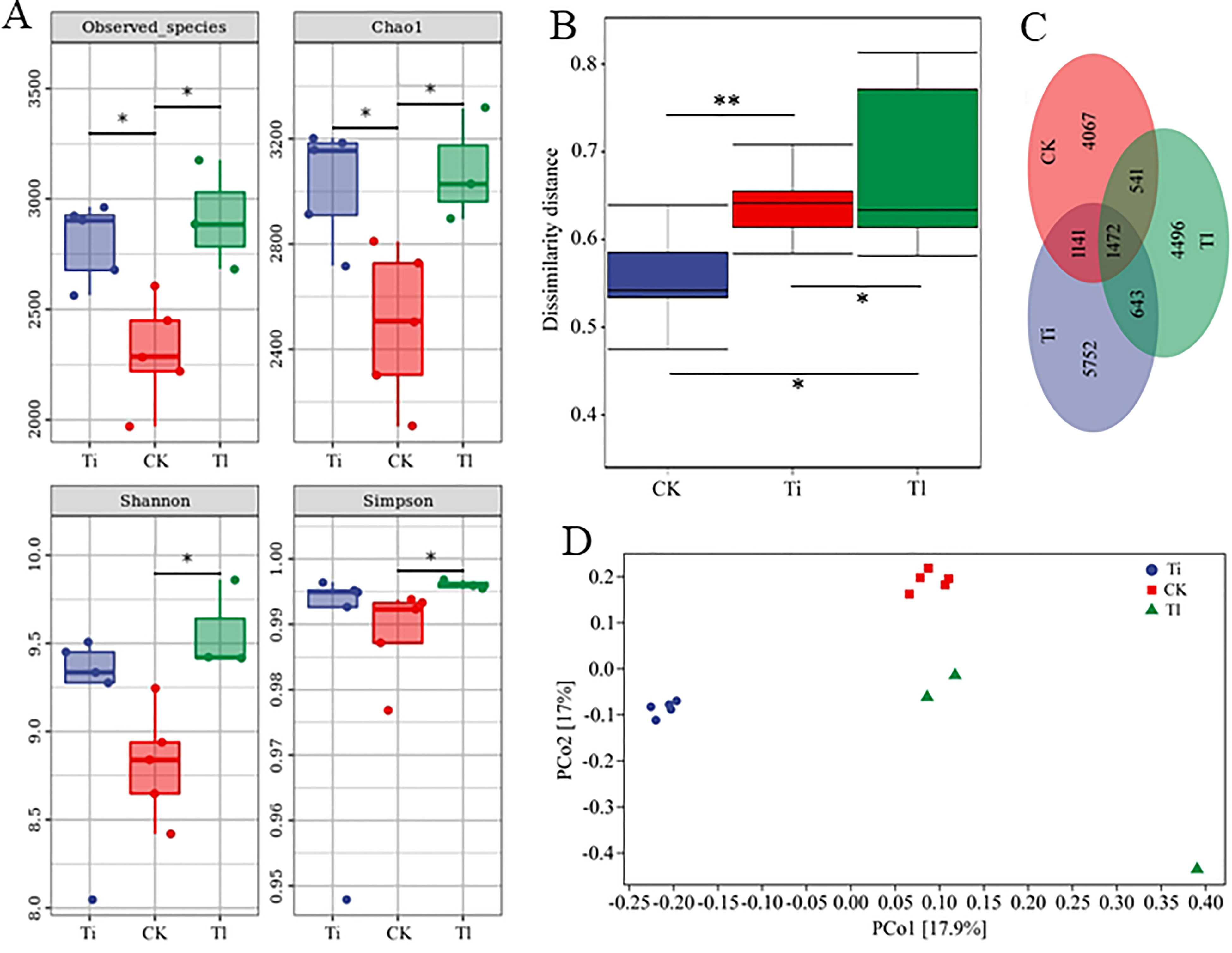
Figure 7 (A–D). Impact of colonization by Tuber indicum (Ti) (n =5) and T. lijiangense (Tl) (n = 3) on Castanopsis rockii seedlings regarding bacterial community richness and diversity (A): Observed species, Chao1 diversity, Shannon index and Simpson index, dissimilarity distance (B), Venn figure showing unique ad operation taxonomic units (OTUs) (C), and principal coordinate analysis (PcoA) (D). CK = control group (n = 5). Significance at P<0.05 *, and P<0.01 **.
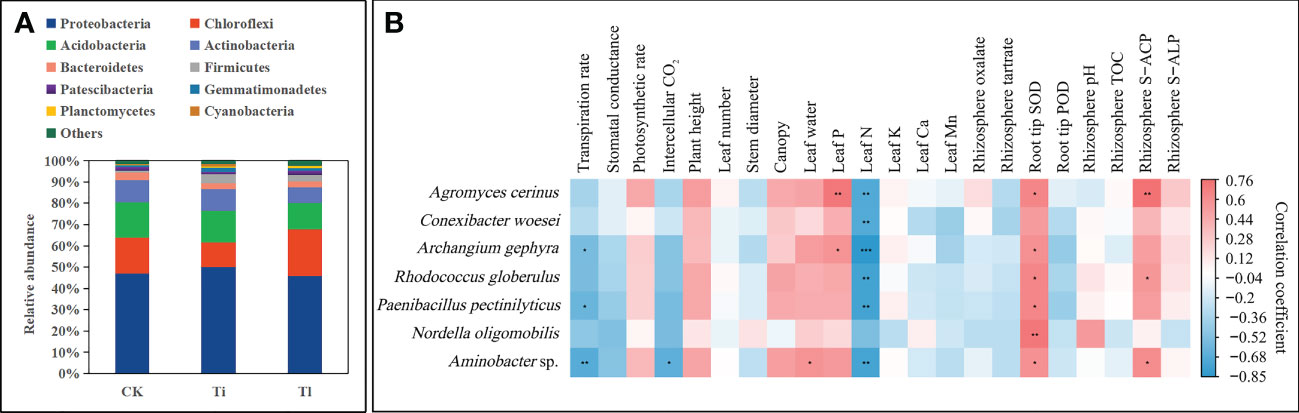
Figure 8 (A, B). Impact of colonization by Tuber indicum (Ti) (n =5) and T. lijiangense (Tl) (n = 3) on Castanopsis rockii seedlings regarding bacterial community structure (A). Heatmap showed the results of Spearman correlation analysis with Bonferroni correction between the 20 most prominent bacterial taxa and plant and soil parameters (B). CK = control group (n = 5). Significance at P<0.05 *, P<0.01 ** and P<0.001 ***.
The 20 most abundant OTUs were selected to perform the Spearman correlation analysis with the experimental plants and soil parameters with the exception of phoC and phoD because the samples used for bacterial analysis were not the same ones as for phoC and phoD gene analysis. Only 7 OTUs (Actinobacteria: Agromyces cerinus, Conexibacter woesei and Rhodococcus globerulus; Proteobacteria: Aminobacter sp., Archangium gephyra, and Nordella oligomobilis; Firmicutes: Paenibacillus pectinilyticus) showed significant relationships with the selected parameters (Figure 8B). Noteworthy, Leaf P was positively correlated with Ag. cerinus and Ar. gephyra. Rhizosphere S-ACP activity was positively correlated with Ag. cerinus, R. globerulus and Aminobacter sp. (Figure 8B).
Discussion
Effects of plant growth and physiological parameters
This work represents the first report of the successful mycorrhizal associations between two Tuber species (T. indicum and T. lijiangense) and C. rockii seedlings. The most important morphological character that allows to distinguish the ectomycorrhizae of the two selected Tuber species is the presence and the shape of the emanating hyphae. T. indicum generally shows woolly or absence of emanating hyphae while T. lijiangense shows abundant spiky emanating hyphae, consistent with previous studies (García-Montero et al., 2008; Wan et al., 2016). The colonization rate of both Tuber species was 50-70% by roughly estimates, but only three out of sixteen seedlings inoculated with T. lijiangense formed mycorrhizae. This different colonization patterns caused strongly different sample sizes. Soil physicochemical properties are an important factor affecting mycorrhizae formation (Bustan et al., 2006; Geng et al., 2009). Considering the fact that the optimal pH for mycelial growth of T. japonicum (white truffle) was 5-6 (Nakano et al, 2020), suggests that a slightly lower initial pH of the substrate might be more suitable for T. lijiangense.
Both fungi significantly enhanced photosynthesis and leaf P concentration of C. rockii, which is consistent with an enhanced photosynthesis and P acquisition in ectomycorrhized Pinus spp. (Reid et al., 1983; Choi et al., 2005), Eucalyptus camaldulensis (Dixon and Hiol-Hiol, 1992) and Quercus spp. (Nehls and Plassard, 2018; Wang et al., 2021; Huang et al., 2022). The enhanced photosynthetic rate could lead to an enhancement of C assimilates, and thus plant growth. The photosynthesis-assimilated C might have been allocated to root exudates and fungal tissues (Wang et al., 2021). However, our results did not agree with this trend because it was found that an enhanced photosynthetic rate and P uptake did not significantly increased plant growth.
Effects of mycorrhization on mycorrhizosphere
We observed lower content of organic anions in the mycorrhizosphere of C. rockii seedlings in association with T. indicum compared to those with T. lijiangense and control plants, with the release of tartrate being especially low in case of T. indicum colonization. The pH in the mycorrhizosphere of seedlings inoculated with T. lijiangense was lower than in those inoculated with T. indicum colonization. These colonization is in accordance with the findings by Casarin et al. (2004), who found that mycorrhizosphere pH is reduced by the release of organic anions. It has been reported that ECM colonization decreases root released organic anions (van Scholl et al., 2006; Meier et al., 2013; Wang et al., 2021), although ECM species such as Paxillus involutus and Piloderma croceum increase rhizosphere oxalate exudation, suggesting that the exudation of organic anions depends on fungal taxon (van Scholl et al., 2006). Nakano et al. (2020) demonstrated that the optimal pH for T. japonicum (a white truffle as T. lijiangense) mycelial growth was much lower than that of T. himalayense and T. longispinosum (both black truffles). Whether this trend will be confirmed is a matter of future investigations. The fact that T. lijiangese (white truffle) is capable of lowering rhizopshere pH supports the hypothesis that ECM fungi modify soil property to their benefit (García-Montero et al., 2017).
Phosphorus is a limiting nutrient in many environments, but plants and microbes have evolved various mechanisms for acquiring soil P, including excretion of phosphatase enzymes (Fraser et al., 2017). In this study, we found that these two Tuber species could significantly favor rhizosphere phoC abundance and acid phosphatase activity in the rhizosphere of C. rockii seedlings, combined with an improved leaf P concentration. Ectomycorrhizal fungi regulate phoC-harbouring microbes to mobilize soil organic P to improved plant P acquisition (Ragot et al., 2015; Fraser et al., 2017). However, neither of the two species studied had any effect on rhizosphere phoD abundance and alkaline phosphatase activity, in agreement with the reduction of species diversity and richness of phoD-harbouring bacteria in rhizosphere of P. armandii seedlings colonized by T. melanosporum (Zhang et al., 2020).
It is well known that SOD and POD play an important anti-oxidation and anti-stress physiological function in plant (Bela et al., 2015). Zhang et al. (2019) found T. indicum inoculation improved the SOD activity of Q. acutissima roots but had no obvious effects on the host plant POD activity, which is consistent with our findings on C. rockii. Similar effects on physiological responses of host plants colonized by T. indicum and T. melanosporum have been reported (Zhang et al., 2020). In our study, T. indicum and T. lijiangense showed different influences on root SOD and POD activity.
Effects of mycorrhization on mycorrhizosphere bacterial community
It has been shown that bacterial richness and diversity in the mycorrhizospehere of T. indicum or T. panzhihuanense and T. borchii are significantly higher than those in the non-ectomycorrhizal roots (Li et al., 2018; Li et al., 2019; Yang et al., 2019). In our study, we found that both Tuber species colonization significantly increased the richness (Chao1 and Observed OTU richness) of bacterial communities over the control, while T. lijiangense colonization also significantly enriched the diversity (Shannon and Simpson) of bacterial communities. It was reported that the presence of ectomycorrhizal fungi can alter the soil microbial composition (Li et al., 2018; Wang et al., 2021). In the present study, the microbial community composition in T. indicum, T. lijiangense and control samples show significant differences, revealing that different Tuber species differently shaped bacterial communities. This finding is most likely caused by different fungus-host combinations that provide different rhizosphere niches (Beckers et al., 2017).
Furthermore, we found that Proteobacteria was the dominant phylum in the mycorrhizosphere of both inoculated seedlings and control group, in agreement with results from other studies (Deveau et al., 2016; Wang et al., 2021). The phylum Chloroflexi was significantly enriched by T. lijiangense mycorrhization, while T. indicum colonization significantly enriched Firmicutes in comparison with the control. Some soil Firmicutes have a strong ability to resists dehydration and extreme conditions (Teixeira et al., 2010; Bulgarelli et al., 2013; Trivedi et al., 2020), and Chloroflexi have been reported having nitrification and carbon cycling abilities (Hug et al., 2013; Chu et al., 2015), which could influence the resistance to stress and N nutrition uptake of plant.
Ag. cerinus has been shown to be potassium-solubilizing (Yang et al., 2017). In our study, Ag. cerinus had significantly positive correlation with mycorrhizosphere S-ACP and leaf P concentration, which might imply that this bacterium also has a role in P mobilization.
Conclusions
This study showed that T. indicum and T. lijiangese have different colonization patterns with C. rockii, and they differentially regulate host plant physiological responses and mycorrhizosphere bacterial communities. However, it should be noted that the unbalanced sample size from T. indiucm, T. lijiangense and control group might limit our results, and additional studies using balanced sample are thus needed to reinforce these findings. In our study, mycorrhization with both fungi significantly enhanced leaf photosynthetic rate, promoted P nutrient acquisition and altered mycorrhizosphere bacterial community of host seedlings. Relative abundances of Ag. cerinus positively correlated to mycorrhizosphere acid phosphatase activity and leaf P concentration, and might thus play an important role in soil P mobilization. These results indicate that ECM fungi species can regulate P-cycling and bacterial community structure in the mycorrhizosphere. However, different Tuber species had different influences on mycorrhizosphere organic anions, root SOD and POD activity, as well as bacterial communities. The content of rhizosphere organic anions caused by T. lijiangense mycorrhization was higher than that in T. indicum, and T. indicum colonization caused significantly higher root SOD but significantly lower root POD than T. lijiangense colonization. These results provide insights into a better understanding of P mobilization and utilization of mycorrhizal seedlings, as well as a theoretical basis for field cultivation of truffles. However, results from this present study are from greenhouse conditions. Whether these two ECM fungi have similar effects on field plants needs further explorations.
Data availability statement
The datasets presented in this study can be found in online repositories. The names of the repository/repositories and accession number(s) can be found in the article/supplementary material.
Author contributions
LH carried out the experimentation, data collection, partial data analysis, and wrote the manuscript. YW and YL analyzed data, conceptualized and reviewed the manuscript. YW and FY designed and instructed this study. JY helped with phoC and phoD analysis. SW contributed to ECM fungi materials and reviewed the manuscript, XH, CC, YL and XS revised the manuscript. All authors contributed to the article and approved the submitted version.
Funding
This work was funded by the National Key R&D Program of China (2021YFD1600404), Yunnan High Level Talent Introduction Plans to YW and XH, Yunnan Key Project of Science and Technology (202102AE090034), and the Basic Research Program of Yunnan Province (202101AU070080) as well as National Natural Science Foundation of China (32170302) to YW.
Acknowledgments
Acknowledge support to the Biotechnology Platform, Kunming Institute of Botany, Chinese Academy of Science. We are thankful to Fei Li and Wei Chang for determine the content of nutrient elements.
Conflict of interest
Author XS was employed by Guizhou Kangqunyuan Biotechnology Co., LTD.
The remaining authors declare that the research was conducted in the absence of any commercial or financial relationships that could be construed as a potential conflict of interest.
Publisher’s note
All claims expressed in this article are solely those of the authors and do not necessarily represent those of their affiliated organizations, or those of the publisher, the editors and the reviewers. Any product that may be evaluated in this article, or claim that may be made by its manufacturer, is not guaranteed or endorsed by the publisher.
References
Alvarez-Lafuente, A., Benito-Matias, L. F., Penuelas-Rubira, J. L., Suz, L. M. (2018). Multi-cropping edible truffles and sweet chestnuts: Production of high-quality Castanea sativa seedlings inoculated with Tuber aestivum, its ecotype T. uncinatum, T. brumale, and T. macrosporum. Mycorrhiza 28, 29–38. doi: 10.1007/s00572-017-0805-9
Andres-Alpuente, A., Sanchez, S., Martin, M., Aguirre, Á.J., Barriuso, J. J. (2014). Comparative analysis of different methods for evaluating quality of Quercus ilex seedlings inoculated with Tuber melanosporum. Mycorrhiza 24, 29–37. doi: 10.1007/s00572-014-0563-x
Beckers, B., Op De Beeck, M., Weyens, N., Boerjan, W., Vangronsveld, J. (2017). Structural variability and niche differentiation in the rhizosphere and endosphere bacterial microbiome of field-grown poplar trees. Microbiome 5, 25. doi: 10.1186/s40168-017-0241-2
Bela, K., Horváth, E., Gallé, Á., Szabados, L., Tari, I., Csiszár, J. (2015). Plant glutathione peroxidases: emerging role of the antioxidant enzymes in plant development and stress responses. J. Plant Physiol. 176, 192–201. doi: 10.1016/j.jplph.2014.12.014
Benucci, G. M. N., Bonito, G. M. (2016). The truffle microbiome: species and geography effects on bacteria associated with fruiting bodies of hypogeous pezizales. Microb. Ecol. 72, 4–8. doi: 10.1007/s00248-016-0755-3
Benucci, G. M. N., Bonito, G., Falini, L. B., Bencivenga, M. (2012). Mycorrhization of pecan trees (Carya illinoinensis) with commercial truffle species: Tuber aestivum vittad. and Tuber borchii vittad. Mycorrhiza 22, 383–392. doi: 10.1007/s00572-011-0413-z
Benucci, G. M. N., Lefevre, C., Bonito, G. (2016). Characterizing root-associated fungal communities and soils of Douglas-fir (Pseudotsuga menziesii) stands that naturally produce Oregon white truffles (Tuber oregonense and Tuber gibbosum). Mycorrhiza 26, 367–376. doi: 10.1007/s00572-015-0677-9
Berendsen, R. L., Pieterse, C. M., Bakker, P. A. (2012). The rhizosphere microbiome and plant health. Trends Plant Sci. 17 (8), 478–486. doi: 10.1016/j.tplants.2012.04.001
Bonito, G., Smith, M. E., Nowak, M., Healy, R. A., Guevara, G., Cázares, E., et al. (2013). Historical biogeography and diversification of truffles in the tuberaceae and their newly identified southern hemisphere sister lineage. PloS One 8, e52765. doi: 10.1371/journal.pone.0052765
Bulgarelli, D., Schlaeppi, K., Spaepen, S., Loren, V., van Themaat, E., Schulze-Lefert, P. (2013). Structure and functions of the bacterial microbiota of plants. Annu. Rev. Plant Biol. 64, 807–838. doi: 10.1146/annurev-arplant-050312-120106
Bustan, A., Ventura, Y., Kagan-Zur, V., Roth-Bejierano, N. (2006). Optimized conditions for mycorrhiza formation between the pink rockrose (Cistus incanus) and the blak périgord truffle (Tuber melanosporum). Isr. J. Plant Sci. 54, 87–96. doi: 10.1560/IJPS_54_2_87
Cairney, J. W. G. (2011). Ectomycorrhizal fungi: The symbiotic route to the root for phosphorus in forest soils. Plant Soil 344, 51–71. doi: 10.1007/s11104-011-0731-0
Casarin, V., Plassard, C., Hinsinger, P., Arvieu, J. C. (2004). Quantifcation of ectomycorrhizal fungal effects on the bioavailability and mobilization of soil p in the rhizosphere of Pinus pinaster. New Phytol. 163, 177–185. doi: 10.1111/j.1469-8137.2004.01093.x
Cheuk, M. L., Fischer, G. A. (2021). The impact of climate change on the distribution of Castanopsis (Fagaceae) species in south China and indo-China region. Glob. Ecol. Conserv. 26, e01388. doi: 10.1016/j.gecco.2020.e01388
Choi, D. S., Quoreshi, A. M., Maruyama, Y., Jin, H. O., Koike, T. (2005). Effect of ectomycorrhizal infection on growth and photosynthetic characteristics of Pinus densifora seedlings grown under elevated CO2 concentrations. Photosynthetica 43, 223–229. doi: 10.1007/s11099-005-0037-7
Chu, Z., Wang, K., Li, X., Zhu, M., Zhang, J. (2015). Microbial characterization of aggregates within a one-stage nitritation-anammox system using high-throughput amlicon aequencing. Chem. Eng. J. 262, 41–48. doi: 10.1016/j.cej.2014.09.067
Deflandre, B., Gagné, J. P. (2001). Estimation of dissolved organic carbon (DOC) Concentrations in nanoliter samples using UV spectroscopy. Water Res. 35 (13), 3057–3062. doi: 10.1016/S0043-1354(01)00024-0
Deveau, A., Antony-Babu, S., Le, T. F., Robin, C., Frey-Klett, P., Uroz, S. (2016). Temporal changes of bacterial communities in the Tuber melanosporum ectomycorrhizosphere during ascocarp development. Mycorrhiza 26 (5), 389–399. doi: 10.1007/s00572-015-0679-7
Dixon, R. K., Hiol-Hiol, F. (1992). Gas exchange and photosynthesis of Eucalyptus camaldulensis seedlings inoculated with different ectomycorrhizal symbionts. Plant Soil 147, 143–149. doi: 10.1007/BF00009380
Dong, L. L., Jiang, Z. H., Wei, W. J., Yuan, H. (2009). Geographical distribution of genus Castanopsis in China. Prot. For. Sci. Technol. 5, 67–69. doi: 10.13601/j.issn.1005-5215.2009.05.001
Edgar, R. C. (2010). Search and clustering orders of magnitude faster. Bioinformatics 26, 2460–2461. doi: 10.1093/bioinformatics/btq461
Bertini, L., Rossi, I., Zambonelli, A., Amicucci, A., Sacchi, A., Cecchini, M., et al. (2006). Molecular identification of Tuber magnatum ectomycorrhizae in the field. Microbiol. Res. 161, 59–64. doi: 10.1016/j.micres.2005.06.003
Fan, L., Cao, J. Z., Liu, Y. Y., Li, Y. (2011). Two new species of Tuber from China. Mycotaxon 116, 349–354. doi: 10.5248/116.349
Fraser, T., Lynch, D. H., Entz, M. H., Dunfield, K. E. (2015). Linking alkaline phosphatase activity with bacterial phoD gene abundance in soil from a long-term management trial. Geoderma 257–258, 115–122. doi: 10.1016/j.geoderma.2014.10.016
Fraser, T. D., Lynch, D. H., Gaiero, J., Khosla, K., Dunfield, K. E. (2017). Quantification of bacterial non-specific acid (phoC) and alkaline (phoD) phosphatase genes in bulk and rhizosphere soil from organically managed soybean fields. Appl. Soil Ecol. 111, 48–56. doi: 10.1016/j.apsoil.2016.11.013
Freiberg, J. A., Sulzbacher, M. A., Grebenc, T., Santana, N. A., Schardong, I. S., Marozzi, G., et al. (2021). Mycorrhization of pecans with European truffles (Tuber spp. tuberaceae) under southern subtropical conditions. Appl. Soil Ecol. 168, 104108. doi: 10.1016/j.apsoil.2021.104108
García-Montero, L., Di Massimo, G., Manjon, J. L., Garcia-Abril, A. (2008). New data onectomycorrhizae and soils of the Chinese truffles Tuber pseudoexcavatum and Tuber indicum, and their impact on truffle cultivation. Mycorrhiza 19, 7–14. doi: 10.1007/s00572-008-0198-x
García-Montero, L. G., Manzano, P., Alwanney, D., Valverde-Asenjo, I., Álvarez-Lafuente, A., Benito-Matías, L. F., et al. (2017). Towards integrated understanding of the rhizosphere phenomenon as ecological driver: Can rhizoculture improve agricultural and forestry systems? In Soil Biol. Communities Ecosystem Resilience pp, 43–75). doi: 10.1007/978-3-319-63336-7_4
Gardes, M., Bruns, T. D. (1993). ITS primers with enhanced specificity for basidiomycetes application to the identifcation of mycorrhizae and rusts. Mol. Ecol. Resour. 2, 113–118. doi: 10.1111/j.1365-294X.1993.tb00005.x
Geng, L. Y., Wang, X. H., Yu, F. Q., Deng, X. J., Tian, X. F., Shi, X. F., et al. (2009). Mycorrhizal synthesis of Tuber indicum with two indigenous hosts, Castanea mollissima and Pinus armandii. Mycorrhiza 19, 461–467. doi: 10.1007/s00572-009-0247-0
Huang, L. L., Guerin-Laguette, A., Wang, R., Li, Y. M., Yu, F. Q. (2021). Mycorrhizal synthesis of Tuber pseudohimalayense with seven broadleaved trees and Pinus armandii. Mycoscience 62, 322–330. doi: 10.47371/mycosci.2021.06.004
Huang, L. L., Guerin-Laguette, A., Wang, R., Yu, F. Q. (2020). Characterization of Tuber indicum (Pezizales, tuberaceae) mycorrhizae synthesized with four host trees exotic to China. Symbiosis 82, 215–224. doi: 10.1007/s13199-020-00715-7
Huang, L. L., Wang, Y. L., Guerin-Laguette, A., Wang, R., Zhang, P., Li, Y. M., et al. (2022). Ectomycorrhizal synthesis between two Tuber species and six tree species: are different host-fungus combinations having dissimilar impacts on host plant growth? Mycorrhiza 32, 341–351. doi: 10.1007/s00572-022-01081-6
Hug, L. A., Castelle, C. J., Wrighton, K. C., Thomas, B. C., Sharon, I., Frischkorn, K. R., et al. (2013). Community genomic analyses constrain the distribution of metabolic traits across the chloroflexi phylum and indicate roles in sediment carbon cycling. Microbiome 1, 22. doi: 10.1186/2049-2618-1-22
Izumi, H., Anderson, L. C., Alexander, I. J., Killham, K., Moore, E. R. B. (2006). Endobacteria in some ectomycoeehiza of scots pine (Pinus sylvestris). FEMS Microbiol. Ecol. 56, 34–43. doi: 10.1111/j.1574-6941.2005.00048.x
Lancellotti, E., Iotti, M., Zambonelli, A., Franceschini, A. (2016). “The puberulum group sensu lato (Whitish truffles),” in True truffle (Tuber spp.) in the world. soil biology, vol 47. Eds. Zambonelli, A., Iotti, M., Murat, C. (Springer, Cham). doi: 10.1007/978-3-319-31436-5_7
Leonardi, M., Iotti, M., Oddis, M., Lalli, G., Pacioni, G., Leonardi, P., et al. (2013). Assessment of ectomycorrhizal fungal communities in the natural habitats of Tuber magnatum (Ascomycota, pezizales). Mycorrhiza 23, 349–358. doi: 10.1007/s00572-012-0474-7
Le Tacon, F. (2016). “Influence of climate on natural distribution of tuber species and truffle production,” in True truffle (Tuber spp.) in the world. soil biology, vol 47. Eds. Zambonelli, A., Iotti, M., Murat, C. (Springer, Cham). doi: 10.1007/978-3-319-31436-5_7
Li, Q., Yan, L., Ye, L., Zhou, J., Zhang, B., Peng, W., et al. (2018). Chinese Black truffle (Tuber indicum) alters the ectomycorrhizosphere and endoectomycosphere microbiome and metabolic profiles of the host tree Quercus aliena. Front. Microbiol. 9. doi: 10.3389/fmicb.2018.02202
Li, X., Zhang, X., Yang, M., Yan, L., Kang, Z., Xiao, Y., et al. (2019). Tuber borchii shapes the ectomycorrhizosphere microbial communities of Corylus avellana. Mycobiology 47 (2), 180–190. doi: 10.1080/12298093.2019.1615297
Liu, P. G., Qiao, P., Zhang, J. P., Zhao, F. L., Zhao, W. Q. (2014). Development prospect of wild edible mushrooms used for ecologic environment conservation and green industry-truffle plantation type used in karst limestone, eco-green, barren hills, mine rehabilitation. Proc. the16th Annu. Meeting China Assoc. Sci. Technol. (Kunming, Yunnan, China), 182–188.
Liu, P. G., Wang, Y., Wang, X. H., Chen, J., Zheng, H. D., Deng, X. J., et al. (2011). Outline of Chinese truffles and their conservational strategies. J. Fungal Res. 9, 232–243.
Mao, L. G., Yan, D. D., Wu, Z. F., Ma, T. T., Wang, Q. X., Li, Y., et al. (2013). Research progress in seed treatment. China Vegetables 10, 9–15.
Marjanović, Z., Nawaz, A., Stevanović, K., Saljnikov, E., Maček, I., Oehl, F., et al. (2020). Root-associated mycobiome differentiate between habitats supporting production of different truffle species in Serbian riparian forests. Microorganisms 8, 1331. doi: 10.3390/microorganisms8091331
Marozzi, G., Benucci, G. M. N., Turchetti, B., Massaccesi, L., Baciarelli Falini, L., Bonito, G., et al. (2022). Fungal and bacterial diversity in the Tuber magnatum ecosystem and microbiome. Microb. Ecol. 85, 508–521. doi: 10.1007/s00248-022-02010-y
Marozzi, G., Sánchez, S., Benucci, G. M. N., Bonito, G., Falini, L. B., Albertini, E., et al. (2017). Mycorrhization of pecan (Carya illinoinensis) with black truffles: Tuber melanosporum and Tuber brumale. Mycorrhiza 27 (3), 303–309. doi: 10.1007/s00572-016-0743-y
Meier, I. C., Avis, P. G., Phillips, R. P. (2013). Fungal communities influence root exudation rates in pine seedlings. FEMS Microbiol. Ecol. 83, 585–595. doi: 10.1111/1574-6941.12016
Mello, A., Balestrini, R. (2018). Recent insights on biological and ecological aspects of ectomycorrhizal fungi and their interactions. Front. Microbiol. 9. doi: 10.3389/fmicb.2018.00216
Mello, A., Murat, C., Bonfante, P. (2006). Truffles: much more than a prized and local fungal delicacy. FEMS Microbiol. Lett. 260, 1–8. doi: 10.1111/j.1574-6968.2006.00252.x
Murat, C. (2015). Forty years of inoculating seedlings with truffle fungi: past and future perspectives. Mycorrhiza 25, 77–81. doi: 10.1007/s00572-014-0593-4
Murat, C., Zampieri, E., Vizzini, A., Bonfante, P. (2008). Is the périgord black truffle threatened by an invasive species? we dreaded it and it has happened! New Phytol. 178, 699–702. doi: 10.1111/j.1469-8137.2008.02449.x
Nakano, S., Kinoshita, A., Obase, K., Nakamura, N., Furusawa, H., Noguchi, K., et al. (2020). Influence of pH on in vitro mycelial growth in three Japanese truffle species: Tuber japonicum, T. himalayense, and T. longispinosum. Mycoscience 61, 58–61. doi: 10.1016/j.myc.2019.12.001
Nehls, U., Plassard, C. (2018). Nitrogen and phosphate metabolism in ectomycorrhizas. New Phytol. 220, 1047–1058. doi: 10.1111/nph.15257
Rácz, A., Hideg, É., Czégény, G. (2018). Selective responses of class III plant peroxidase isoforms to environmentally relevant UV-b doses. J. Plant Physiol. 221, 101–106. doi: 10.1016/j.jplph.2017.12.010
Ragot, S. A., Kertesz, M. A., Bünemann, E. K. (2015). phoD alkaline phosphatase gene diversity in soil. Appl. Environ. Microbiol. 81 (20), 7281–7289. doi: 10.1128/AEM.01823-15
Reid, C. P. P., Kidd, F. A., Ekwebelam, S. A. (1983). Nitrogen nutrition, photosynthesis and carbon allocation in ectomycorrhizal pine. Plant Soil 71, 415–431. doi: 10.1007/BF02182683
Ren, H., Yang, L., Liu, N. (2008). Nurse plant theory and its application in ecological restoration in lower subtropics of China. Prog. Nat. Sci. 16, 137–142. doi: 10.1016/j.pnsc.2007.07.008
Shafi, A., Chauhan, R., Gill, T., Swarnkar, M. K., Sreenivasulu, Y., Kumar, S., et al. (2015). Expression of SOD and APX genes positively regulates secondary cell wall biosynthesis and promotes plant growth and yield in Arabidopsis under salt stress. Plant Mol. Biol. 87 (6), 615–631. doi: 10.1007/s11103-015-0301-6
Smith, S. E., Read, D. J. (2008). Mycorrhizal symbiosis. 3rd edition (Academic, New York: Academic Press, London).
Teixeira, L. C. R. S., Peixoto, R. S., Cury, J. C., Sul, W. J., Pellizari, V. H., Tiedje, J., et al. (2010). Bacterial diversity in rhizosphere soil from Antarctic vascular plants of admiralty bay, maritime Antarctica. ISME J. 4, 989–1001. doi: 10.1038/ismej.2010.35
Trappe, J. M., Molina, R., Luoma, D., Cázares, E., Pilz, D., Smith, J. E., et al. (2009). Diversity, ecology, and conservation of truffle fungi in forests of the pacific Northwest. Ben. Tech. Rep. (Portland), 194p. doi: 10.2737/PNW-GTR-772
Trivedi, P., Leach, J. E., Tringe, S. G., Sa, T., Singh, B. K. (2020). Plant–microbiome interactions: from community assembly to plant health. Nat. Rev. Microbiol. 18, 607–621. doi: 10.1038/s41579-020-0412-1
van der Heijden, M. G. A., Martin, F. M., Selosse, M. A., Sanders, I. R. (2015). Mycorrhizal ecology and evolution: the past, the present, and the future. New Phytol. 205, 1406–1423. doi: 10.1111/nph.13288
van Scholl, L., Hofand, E., van Breemen, N. (2006). Organic anion exudation by ectomycorrhizal fungi and Pinus sylvestris in response to nutrient deficiencies. New Phytol. 170, 153–163. doi: 10.1111/j.1469-8137.2006.01649.x
Wan, S. P., Yu, F. Q., Tang, L., Wang, R., Wang, Y., Liu, P. G., et al. (2016). Ectomycorrhizae of Tuber huidongense and T. liyuanum with Castanea mollissima and Pinus armandii. Mycorrhiza 26, 249–256. doi: 10.1007/s00572-015-0663-2
Wang, Y., Lambers, H. (2020). Root-released organic anions in response to low phosphorus availability: recent progress, challenges and future perspectives. Plant Soil 447, 135–156. doi: 10.1007/s11104-019-03972-8
Wang, Y., Wang, R., Lu, B., Guerin-Laguette, A., He, X., Yu, F. (2021). Mycorrhization of Quercus mongolica seedlings by Tuber melanosporum alters root carbon exudation and rhizosphere bacterial communities. Plant Soil 467, 391–403. doi: 10.1007/s11104-021-05112-7
Wang, Y., Yu, F. Q., Zhang, C. X., Liu, C. Y., Yang, M., Li, S. H. (2020). “Edible ectomycorrhizal fungi and their cultivation in China,” in Mushrooms, humans and nature in a changing world, perspective from ecological agricultural and social science. Eds. Pérez-Moreno, J., Guerin-Laguette, A., Flores-Arzú, R., Yu, F. Q. (Springer, Cham), pp 31–pp 60. doi: 10.1007/978-3-030-37378-8_2
White, T. J., Bruns, T., Lee, S., Taylor, J. W. (1990). “Amplification and direct sequencing of fungal ribosomal RNA genes for phylogenetics,”. Eds. Innis, M. A., Gelfand, D. H., Sninsky, J. J., White, T. J. (San Diego: PCR Protocols. Academic Press), 315–322.
Yang, Q., Yao, Q., Yuan, Z., Yan, W. (2017). Screening and identification of phosphate and potassium dissolving bacteria from mycorrhizosphere of Pinus tabuliformis. Genomics Appl. Biol. 36 (4), 1526–1531.
Yang, M., Zou, J., Liu, C., Xiao, Y., Zhang, X., Yan, L., et al. (2019). Chinese White truffles shape the ectomycorrhizal microbial communities of Corylus avellana. Ann. Microbiol. 69, 553–565. doi: 10.1007/s13213-019-1445-4
Zhang, X., Li, X., Ye, L., Huang, Y., Kang, Z., Zhang, B., et al. (2020). Colonization by Tuber melanosporum and Tuber indicum affects the growth of Pinus armandii and phoD alkaline phosphatase encoding bacterial community in the rhizosphere. Microbiol. Res. 239, 126520. doi: 10.1016/j.micres.2020.126520
Keywords: ectomycorrhiza, nutrient acquisition, phoC and phoD, phosphatase activity, rhizosphere exudates, mycorrhizosphere microbiome
Citation: Huang L, Li Y, Yuan J, Wan S, Colinas C, He X, Shi X, Wang Y and Yu F (2023) Tuber indicum and T. lijiangense colonization differentially regulates plant physiological responses and mycorrhizosphere bacterial community of Castanopsis rockii seedlings. Front. Plant Sci. 14:1134446. doi: 10.3389/fpls.2023.1134446
Received: 30 December 2022; Accepted: 28 March 2023;
Published: 12 April 2023.
Edited by:
Rutger A. Wilschut, Wageningen University and Research, NetherlandsReviewed by:
Serena Santolamazza, University of Vigo, SpainKoshila Ravi Ravichandran, Bharathiar University, India
Copyright © 2023 Huang, Li, Yuan, Wan, Colinas, He, Shi, Wang and Yu. This is an open-access article distributed under the terms of the Creative Commons Attribution License (CC BY). The use, distribution or reproduction in other forums is permitted, provided the original author(s) and the copyright owner(s) are credited and that the original publication in this journal is cited, in accordance with accepted academic practice. No use, distribution or reproduction is permitted which does not comply with these terms.
*Correspondence: Yanliang Wang, wangyanliang@mail.kib.ac.cn; Fuqiang Yu, fqyu@mail.kib.ac.cn
†These authors have contributed equally to this work