Maintaining the protective function of mountain forests under climate change by the concept of naturalness in tree species composition
- 1Plant Regeneration Ecology, Forest Resources and Management, Swiss Federal Institute for Forest, Snow and Landscape Research WSL, Birmensdorf, Switzerland
- 2Scientific Service NFI, Forest Resources and Management, Swiss Federal Institute for Forest, Snow and Landscape Research WSL, Birmensdorf, Switzerland
- 3Silviculture Group, ETH Zürich, Zürich, Switzerland
- 4Forest Dynamics, Swiss Federal Institute for Forest, Snow and Landscape Research WSL, Birmensdorf, Switzerland
In Swiss mountain areas, the protective function of forests is the predominant ecosystem service having high cultural and economic significance. It is assumed that natural forests or close-to-natural forests, i.e., forests being in the equilibrium with environmental conditions are the most resilient and resistant in regard to disturbances and hence best protecting people and assets on the long run. Here, we estimated the naturalness of the tree species composition by comparing Swiss National Forest Inventory (NFI) data with current and future potential Natural forest Site Types (NST). Based on this analysis, we identified species that are under or over-represented in protective mountain forests and derived the subsequent potential for management interventions. The urgency of management interventions is expected be small if all predominant tree species of the idealized potential natural forests are present and only their relative portions in the stand need adjustment. In contrast, interventions are advisable, if predominant tree species of the current and future potential natural forests are absent. Based on NFI data, the tree species composition of 47% of the protective mountain forests were classified as “natural” or “close-to-natural,” while the remaining 53% were classified as “not natural” or “partly natural.” Norway spruce [Picea abies (L.) H. Karst.] and European larch (Larix decidua Mill.) were the two most over-represented species under current and even more so under predicted future climatic conditions. To date, silver fir (Abies alba Mill.) and European beech (Fagus sylvatica L.) were the two species most frequently absent in protective mountain forests, in which they should prevail. Apart from European beech, the most prominent increase in prevalence is predicted for oak (sessile oak and pubescent oak; Quercus petrea Liebl., Q. pubescens Willd.) and small-leaved lime (Tilia cordata Mill.). These species were currently missing from more than 75% of the stands, in which they are expected to be dominant under future conditions. Our analysis indicates the need to transform tree species compositions of protective mountain forests to optimize fitness under future climates. Some of these transformations will take place naturally, incited by disturbances, others—the majority of them—will need active management interventions.
Introduction
Forests in mountain areas provide several ecosystem services (Ninan, 2014; Acharya et al., 2019) including protection for critical infrastructure such as roads, railroads, and buildings resulting in considerable economic and cultural value (Teich and Bebi, 2009; Miura et al., 2015). In Switzerland about 50% of mountain forests protect 130,000 buildings and several thousand kilometers of traffic routes generating an estimated ecosystem service value of 4 bn $ per year (Losey and Wehrli, 2013). Continued protective function is therefore essential to further mitigate socio-economic risks.
It is generally assumed that forests/ecosystems in equilibrium with the environmental conditions (i.e., natural forests) are most stable and consequently best suited to provide continued ecosystem services (Stritih et al., 2021; Scherrer et al., 2023a). As ongoing climate change affects tree habitat ranges and community compositions (e.g., Dyderski et al., 2018; Lenoir et al., 2020) these protective forests should necessarily adapt to stay in equilibrium with their dominant environmental conditions (Brang et al., 2014; Albrich et al., 2018). This poses several challenges. Firstly, forest ecosystems transform very slowly with environmental change, especially in the absence of stand-level disturbances (Thom et al., 2017; Scherrer et al., 2020, 2022). Secondly, after a large-scale disturbance, forests go through a decade-long series of successional stages before reaching a new climax state (Breshears et al., 2011; Seidl et al., 2017). And finally, most forests in Switzerland are highly managed systems with a long land-use history leading to differing degrees of naturalness (Scherrer et al., 2023a). A steady adaptation of mountain forests by management is therefore necessary to maintain the suitability of forests to environmental conditions and ensure a continued protective function (Pluess et al., 2016; Daniel et al., 2017; Runting et al., 2017). The type and intensity of management intervention needed to promote a high naturalness in future mountain forests strongly depends on its the current degree of naturalness.
In Switzerland, forest management practices aim at creating more resilient, “climate-smart” forests (e.g., Bowditch et al., 2020; Mathys et al., 2021; Santopuoli et al., 2021) ensuring continued ecosystem services (e.g., Nabuurs et al., 2017; Temperli et al., 2020; Verkerk et al., 2020). Many Swiss forests are currently managed by a “close-to-nature” silviculture system (Bürgi, 2015; Spathelf et al., 2015) favoring natural regeneration (Brändli et al., 2020b) in small gaps by direct regrowth. An important aspect of “close-to-nature” silviculture is to create forests adapting to changing environmental conditions due to increased naturalness (Brang et al., 2014; Spathelf et al., 2015). In line with this idea, the Swiss Forest Act (WaG, SR 921.0) states that tree recruitment or planting should be based on site-adapted species (i.e., species of the potential natural forest community). Consequently, information about the potential natural forest community has been provided for different cantons and regions, and an integral classification system provides now comparability nationwide: the NaiS [Nachhaltigkeit und Erfolgskontrolle im Schutzwald (Sustainability in the Protection Forest); Frehner et al., 2009; Frey et al., 2021] natural forest site system. The Natural forest Site Types (NST; i.e., idealised potential natural vegetation with characteristic tree species composition; e.g., Prentice et al., 1992; Koca et al., 2006) of a given location is determined by expert knowledge based on abiotic conditions (e.g., elevation, geographic region, soil water availability and pH), site/topographic factors (e.g., big boulders, streams, avalanche tracks) as well as by the composition and growth of the understory vegetation. A NST provides several parameters used to judge the protective function of a forest stand, including the potential natural tree species composition. The naturalness of a forest stand can, therefore, be determined by comparing the observed tree species composition with the “potential,” i.e., assumed natural, tree composition of an NST. As the composition of the canopy trees is defined by both, site conditions and former/current management, naturalness might give a good indication of the current and future need for management interventions to ensure optimal forest stability (i.e., high naturalness).
In this study, we used data from the 4th Swiss National Forest Inventory (NFI4; 2009–2017) in combination with information on the current and projected future NST (1) to evaluate the current and projected future naturalness of protective mountain forests, (2) to identify tree species that are accordingly over-, underrepresented or even missing from forest stands where they are expected to prevail and (3) to determine the indicated need for forest management interventions to ensure continued protective function under climate change.
Materials and methods
Idealized natural forest compositions according to NST
In Switzerland the quality of protective forests is evaluated based on the NaiS-System (Frehner et al., 2005). The NaiS-System evolved from the old Swiss forest classification system of Ellenberg and Klötzli (1972) and provides information about the NST, as well as associated minimal or ideal stand requirements for the protective function (e.g., number of stems, gap sizes, crown length; Frehner et al., 2005). We focused on the NST’s assumed natural tree species composition that is indicated by the floristic composition of the herbaceous layer, site quality and structural characteristics. NSTs depict the tree species composition (including recruitment) in an idealized natural forest at the optimum developmental stage that is considered being in balance with the dominant environmental conditions (e.g., climate, topography, soil, canopy closure; Ott et al., 1997; Frey et al., 2021). Currently, there are more than 433 NSTs defined for Switzerland, each with its own potential natural tree species composition. Based on NFI data, 266 of those NSTs are currently recorded in Switzerland and the remaining 167 represent potential new NSTs expected to appear until the end of the century (i.e., according to diverse climate change scenarios; Supplementary Table 1; Frehner et al., 2019). These future potential NST were based on the existing NST and extended with potential new better adapted tree species based on species distribution models (Zimmermann et al., 2014), studies about analog climatic regions (Huber et al., 2017), and species range maps (Schütt et al., 2006; for more details see Frehner et al., 2019). All tree species occurring in Switzerland (N = 75, both native and non-native), are assigned to each of these NSTs, following a classification into four categories (a) “dominant tree species of the idealized natural forest,” (b) “important supplementary tree species of the idealized natural forest,” (c) “additional tree species of the idealized natural forest” and (d) “tree species not part of the idealized natural forest” (Supplementary Table 1). Of the 266 NSTs currently observed in Switzerland, 62% are monodominant (e.g., European beech forests), 32% are co-dominated by two species (e.g., silver fir-Norway spruce forests) and only 6% are co-dominated by more than two species (e.g., oak-lime forests). The proportion of future NSTs with more than two co-dominant species is higher (25%), reflecting the uncertainty in potential species composition under future climatic conditions.
National Forest Inventory data (NFI)
The 4th Swiss National Forest Inventory (NFI4; 2009–2017) was recorded on a 1.4 × 1.4 km systematic permanent sampling grid covering the whole forested area of Switzerland (Brändli et al., 2020a). We restricted the data of NFI plots to the perimeter of protective mountain forests delimitation of the cantons according to the federal office of environment (Losey and Wehrli, 2013; Figure 1). A terrestrial NFI plot assessment consists of numerous elements (Lanz et al., 2019), of which we used the proportion of all canopy-forming tree species (i.e., species-specific area cover in the canopy layer) of the reference stand (i.e., the stand with the plot centre within the 50 × 50 m interpretation area; Fischer and Traub, 2019), the presence of all woody species in a 200 m2 circle around the plot centre, and the assigned current and future Natural forest Site Types (NSTcurrent, NSTRCP4.5 and NSTRCP8.5; ARGE Frehner et al., 2020, Frey et al., 2021) covering the plot centre. The NSTcurrent was defined by experts for 40% of the NFI sample plots in the field and for 60% of the plots by analogy conclusions using topography and factor maps (ARGE Frehner et al., 2020). The future NSTs were determined based on potential shifts in the vegetation belts, resulting from climate warming (Zischg et al., 2021) in combination with local site factors based on existing ecograms (for more details see Frehner et al., 2019) using the website Tree-App.ch.1 Future NSTs were projected for 2085 (average of 2070-2099) under global warming scenarios using two different Representative Concentration Pathways (RCP 4.5 and RCP 8.5) based on the climate projection of MeteoSwiss (CH2018, 2018). Data on the proportion of all canopy-forming tree species was used to infer dominance of species as well as an indication on the availability of seed trees. Data on the presence of woody species was mainly used to determine if a species is present at all, even just as a single seedling. In total, the NFI covered 2,072 plots located in protective forests in mountain regions for which data on the tree species composition and NSTs were available (Figure 1).
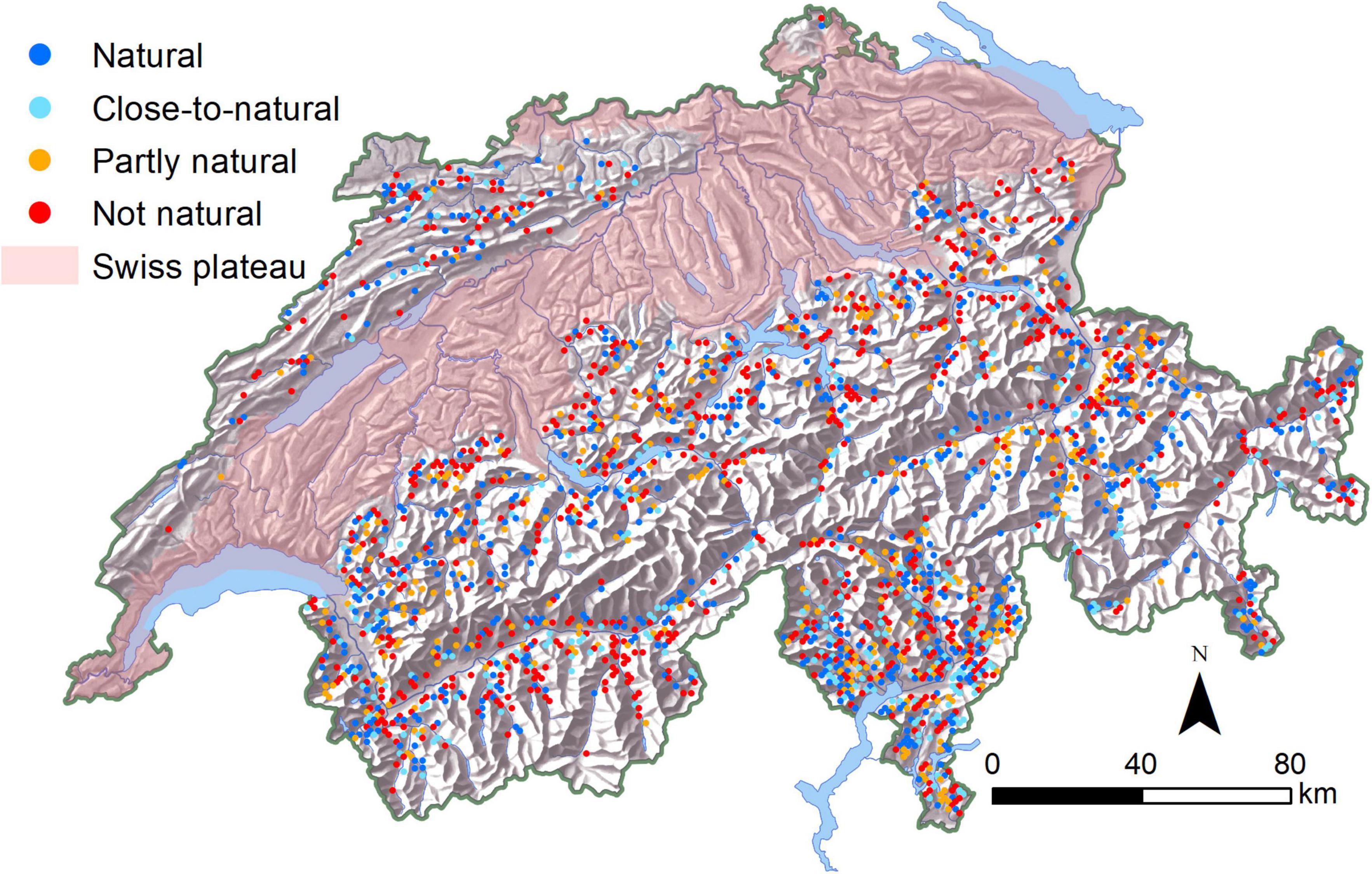
Figure 1. Location and calculated current naturalness (i.e., based on NSTcurrent) of the Swiss National Forest Inventory (NFI) plots located in protective mountain forests.
Naturalness and species composition
The naturalness of a NFI plot was determined by comparing the reported tree species composition of the canopy layer based on NFI data with the natural tree species composition based on the NST of the plot. We distinguished four categories of naturalness: (1) “natural forest,” (2) “close-to-natural forest,” (3) “partly natural forest,” and (4) “not natural forest” based on the criteria of Table 1. All these calculations were done separately for NSTcurrent, NSTRCP4.5, and NSTRCP8.5 but always in comparison with the tree species composition observed during the NFI4 (2009–2017). The classification of NFI plots into the four different categories of naturalness is illustrated by several examples in Supplementary Appendix 1.
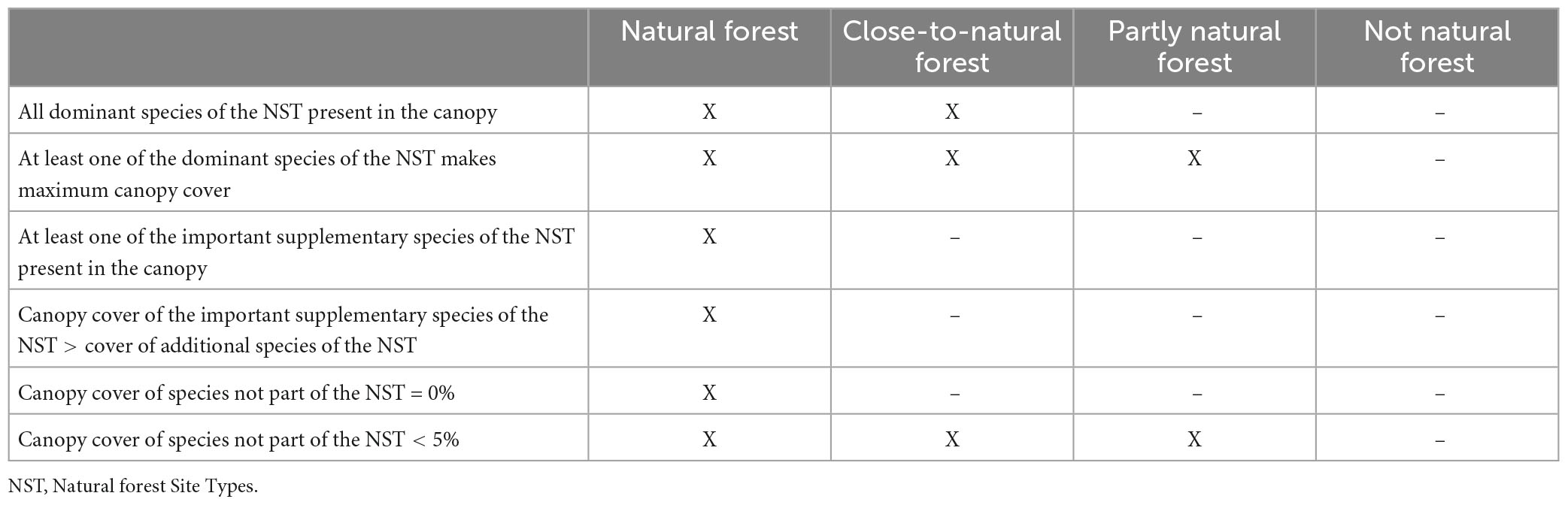
Table 1. Overview of the criteria used to evaluate the naturalness of the tree species composition of a forest stand.
For each NFI plot we determined the tree species that are currently dominant in terms of canopy cover as well as the tree species that should be dominant in an idealized natural forest under current and future conditions (NSTcurrent, NSTRCP4.5, NSTRCP8.5). In addition, we evaluated which tree species are dominant or important supplementary species of the idealized natural forest under current and future conditions but currently missing from the plots. This was calculated (1) based on the canopy cover data (i.e., counting a tree species as absent if not reaching the canopy layer) and (2) based on the presence data of all tree species (i.e., counting a species as present even if present only as a seedling). This allowed us to identify tree species that are under or over-represented in protective forests and to derive the subsequent need for management interventions. The urgency of management intervention might be relatively low, if all predominant tree species of the potential natural forests are present and only their relative portions in the stand need adjustment. Or it might be high, if predominant tree species of the current and future expected natural forest are absent.
Results
Naturalness of Swiss protective mountain forests
Under current conditions, 47% of the NFI plots in protective mountain forests were covered by “natural” or “close-to-natural” tree species compositions while 14 and 39% were classified as “partly natural” and “not natural,” respectively (Figure 1). Without any adaptations (by natural succession or by management), the proportion of NFI plots with “natural” or “close-to-natural” tree species composition would drop to 28 and 19% until 2085 under global warming scenarios RCP 4.5 and RCP 8.5, respectively (Figure 2).
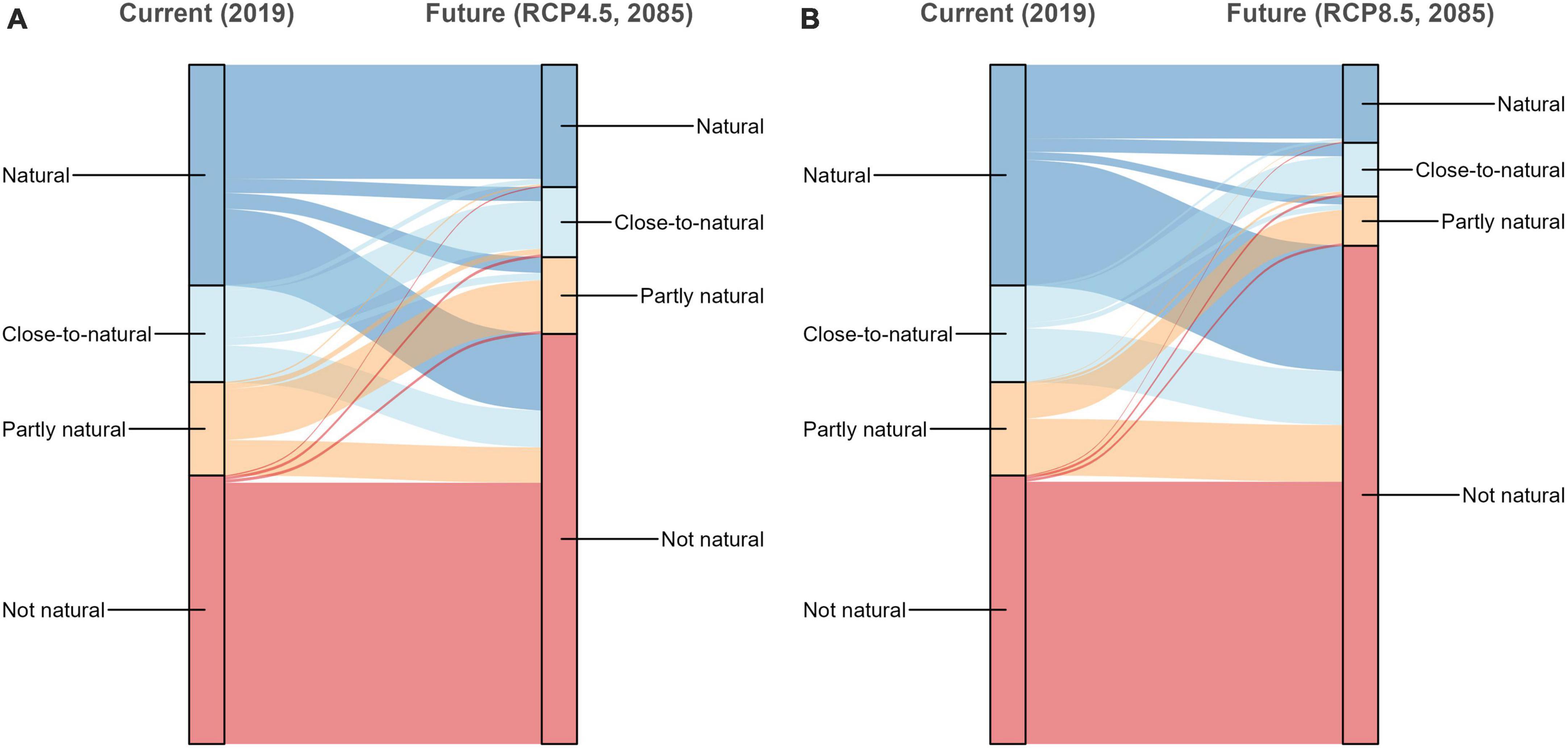
Figure 2. Proportions and development of naturalness indices of Swiss National Forest Inventory (NFI) plots in protective mountain forests under current and future conditions using scenarios RCP 4.5 (A) and RCP 8.5 (B).
Most NFI plots in protective mountain forests were dominated by Norway spruce [48.7%; Picea abies (L.) H. Karst.]. Under current conditions, 48.1% of these Norway spruce dominated NFI plots were considered “natural” or “close-to-natural” (i.e., NST dominated by Norway spruce) and 29.7% “not natural” (Figure 3). Other tree species frequently dominating the NFI plots in protective mountain forests were European beech (22.4%; Fagus sylvatica L.), silver fir (16.3%; Abies alba Mill.), and European larch (15.3%; Larix decidua Mill.) which, under current conditions, were considered “natural” or “close-to-natural” in 67.9, 57.8, 19.8, and “not natural” in 17.9, 31.2, 61.5%, respectively (Figure 3). Assuming no changes in tree species composition, their naturalness was strongly decreasing under future conditions (Figure 3 and Supplementary Figure 1). In fact, only NFI plots currently dominated by sessile oak (1.25%; Quercus petraea Liebl.) and small-leaved lime (0.8%; Tilia cordata Mill.) were projected to increase in naturalness under future conditions (i.e., they had a tree species composition better fitting expected future than current site conditions; Figure 3 and Supplementary Figure 1).
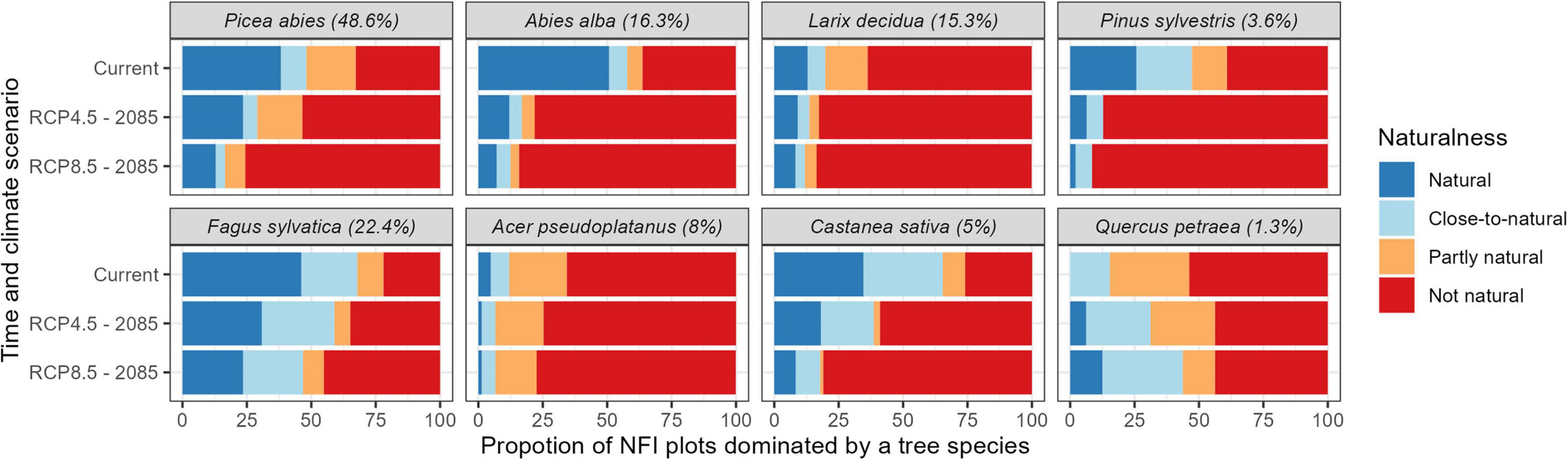
Figure 3. Naturalness of National Forest Inventory (NFI) plots dominated or co-dominated by the principal four conifer (top) and four broadleaf species (bottom) in protective mountain forests under current and future climatic conditions assuming no changes in species composition. The numbers in the brackets indicate the proportion of protection forests currently (co-)dominated by the species.
Dominant and lacking species in Swiss protective mountain forests
The decrease in naturalness under future climatic conditions corresponded with the expected changes in NSTs of protective mountain forests (Figure 4). Currently, protective forests should be dominated by Norway spruce, European beech, and silver fir (Figure 4). In the future, the dominance of the most frequent conifers was predicted to strongly decrease while the proportion of European beech dominated NFI plots remained rather constant. Many other broadleaf species were predicted to increase within protective forests, especially oak (sessile oak and pubescent oak; Quercus petrea Liebl., Q. pubescens Willd.) and lime (Tilia spp.; Figure 4 and Supplementary Figure 2).
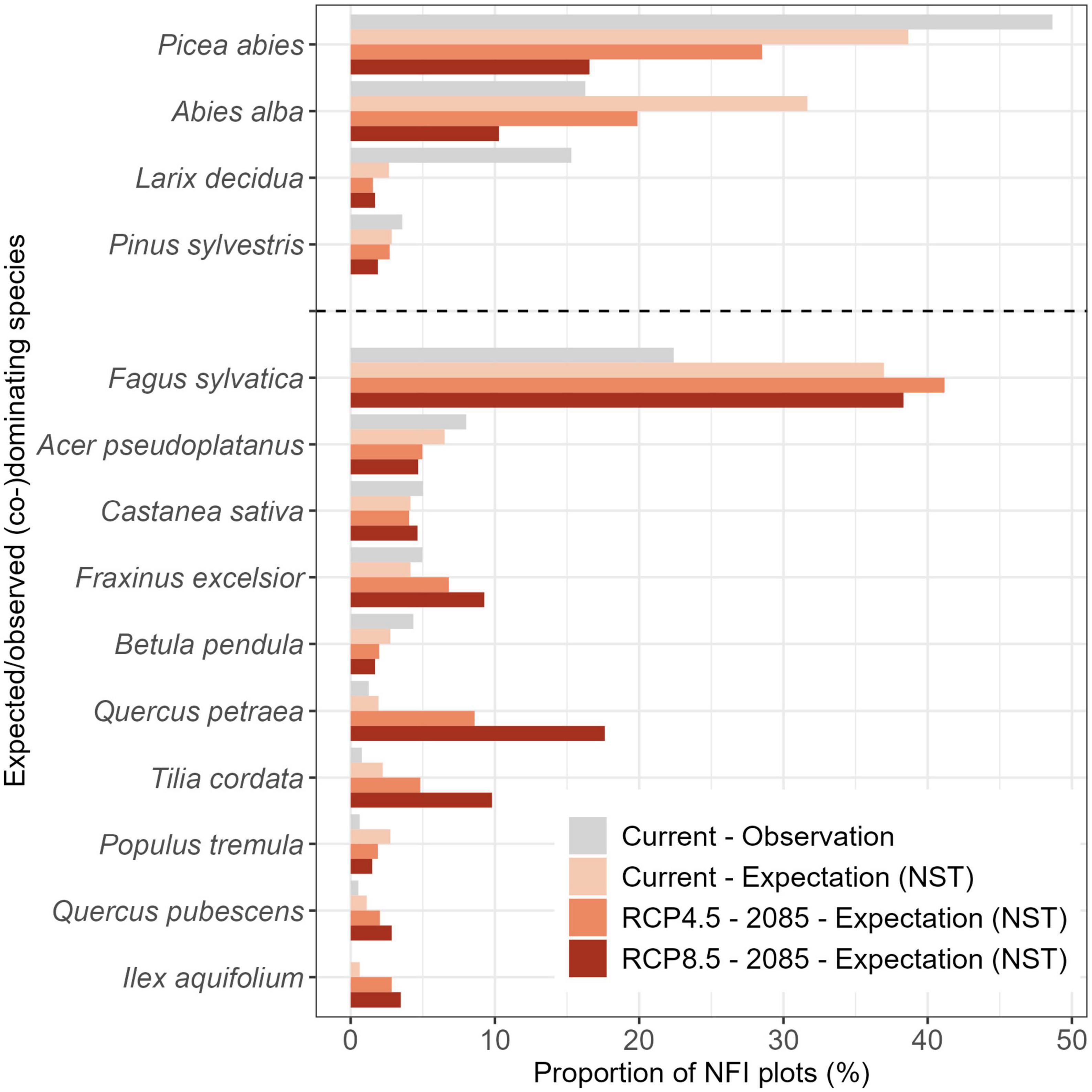
Figure 4. Tree species that were currently (co-)dominating the NFI plots in protective mountain forests (gray) or were expected to (co-)dominate based on the Natural forest Site Types (NST) under current and future climatic conditions in 2085 (shades of red). Species are separated into conifers (top) and broadleaves (bottom). Only species that (co-)dominated at least 2.5% of the NFI plots in protective mountain forests are shown (for all species see Supplementary Figure 2).
Norway spruce and European larch were the two most over-represented species (i.e., species dominating NFI plots where other species should be dominant according to the NST) under current and even more so under predicted future conditions (Figure 5). In general, the over-representation of conifers was predicted to increase while the over-representation of broadleaf species was rather constant with the notable exceptions of European beech and sweet chestnut (Castanea sativa Mill.; Figure 5). This indicates that while the proportion of NFI plots dominated by European beech and sweet chestnut are rather constant across time (Figure 4), the location of plots dominated by those species will shift in elevation.
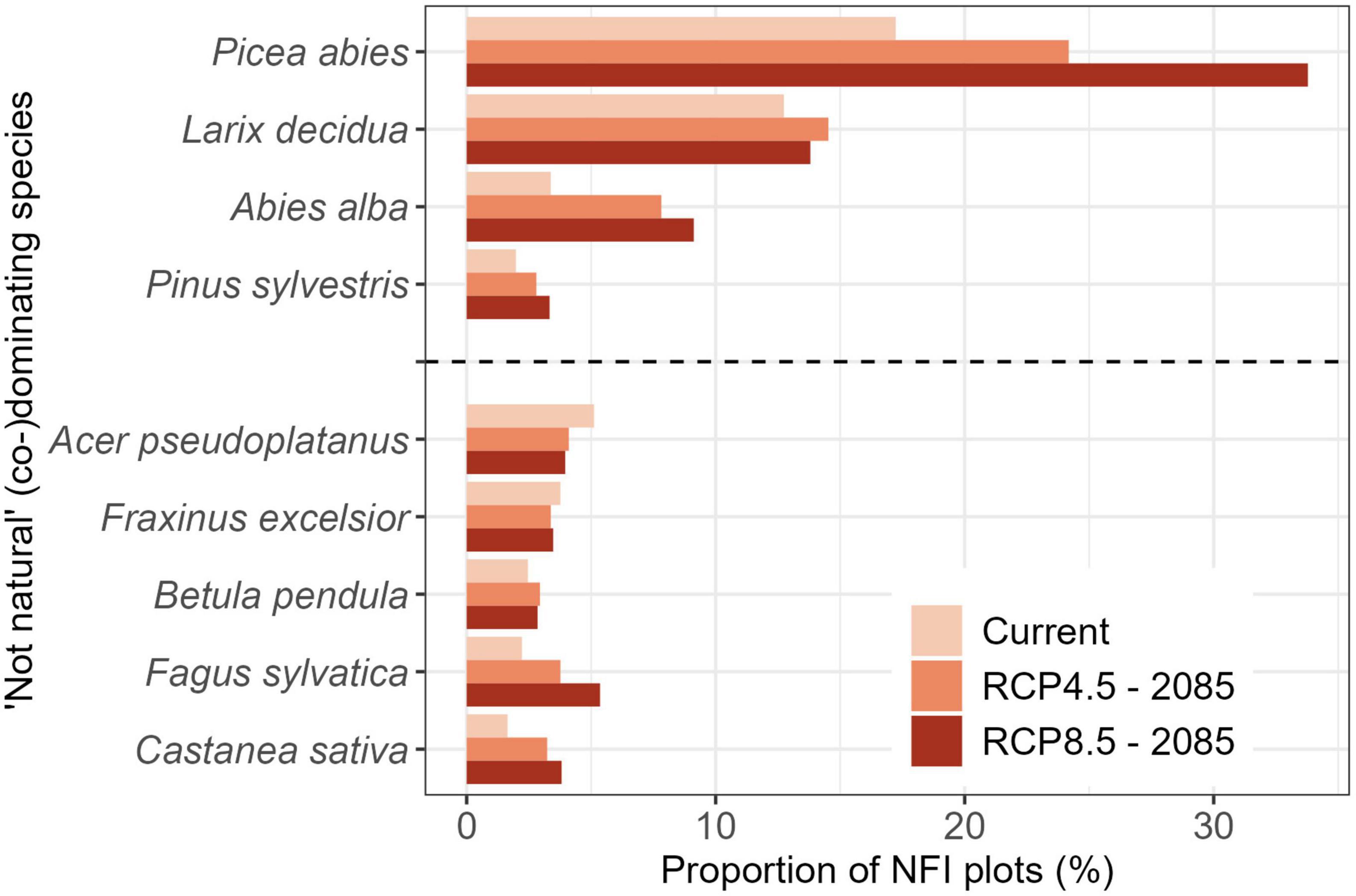
Figure 5. Proportion National Forest Inventory (NFI) plots currently (co-)dominated by a tree species not matching the current or future Natural forest Site Types (NST). Species are separated into conifers (top) and broadleaves (bottom). Only species mismatches that occurred on more than 2.5% of the NFI plots in protective mountain forests are shown (for all species see Supplementary Figure 3).
Regarding the latest NFI inventory, silver fir and European beech were the two species most frequently absent from NFI plots, even though they would dominate under natural conditions. This held for both, the canopy layer (i.e., seed trees) and in general (i.e., not even a seedling present; Figure 6 and Supplementary Figure 5). In the case of silver fir, the expected presence as dominant species should decrease due to adverse climatic conditions in the future. The importance of European beech will further increase by proportions, as a result of extended suitability toward higher elevations where the species would prevail in the future but was currently missing in the last NFI inventory (Figure 6). Many broadleaf species such as oak, small-leaved lime, ash (Fraxinus excelsior L), holly (Ilex aquifolium L.) and sweet chestnut were rarely (co-)dominating, but their importance was predicted to drastically increase under future climatic conditions (Figure 6). These broadleaf species were currently missing from the majority (≥50%) of NFI plots, where they are expected to be present and eventually prevailing under future conditions, according to the NSTRCP4.5 and NSTRCP8.5 (Supplementary Table 2).
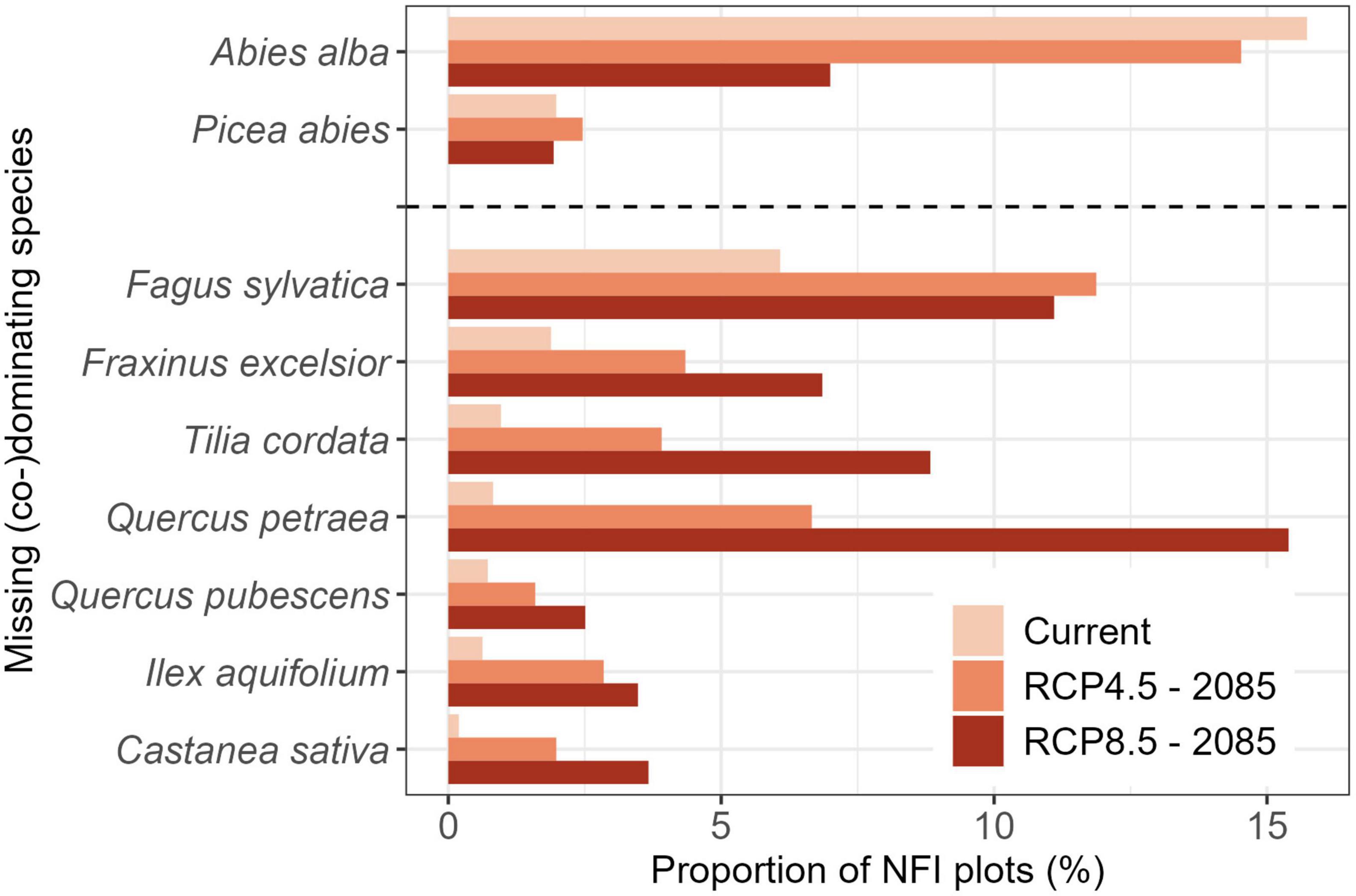
Figure 6. Proportion of National Forest Inventory (NFI) plots with canopy layers lacking a tree species that should be (co-)dominating the current or future protective mountain forest stands based on the Natural forest Site Types (NST). Species are separated into conifers (top) and broadleaves (bottom). Only species missing from more than 2.5% of the NFI plots in protective mountain forests are shown (for all species see Supplementary Figures 4, 5).
In the inventory, Sorbus spp. (rowan and whitebeam; Sorbus aucuparia L. and Sorbus aria (L.) Crantz), ash, sycamore (Acer pseudoplatanus L.) and elm (Ulmus glabra Huds.) were the important supplementary species of the idealized natural forest that were most frequently missing from NFI plots according to the NSTcurrent (Figure 7). Under future conditions (NSTRCP4.5 and NSTRCP8.5), the species most frequently missing as important supplementary species of the idealized natural forests were projected to be Quercus spp. (Q. petraea, Q. robur, Q. pubescens, Q. cerris), Acer spp. (A. pseudoplatanus, A. platanoides, A. campestre, A. opalus), Tilia spp. (T. cordata, T. platyphyllos), wild cherry (Prunus avium L.) and ash (Figure 7). Like the dominant species, these future broadleaf species were currently completely missing in the inventories (i.e., not even present as seedlings) where they are expected to be important elements of the future protective mountain forests (Supplementary Table 3).
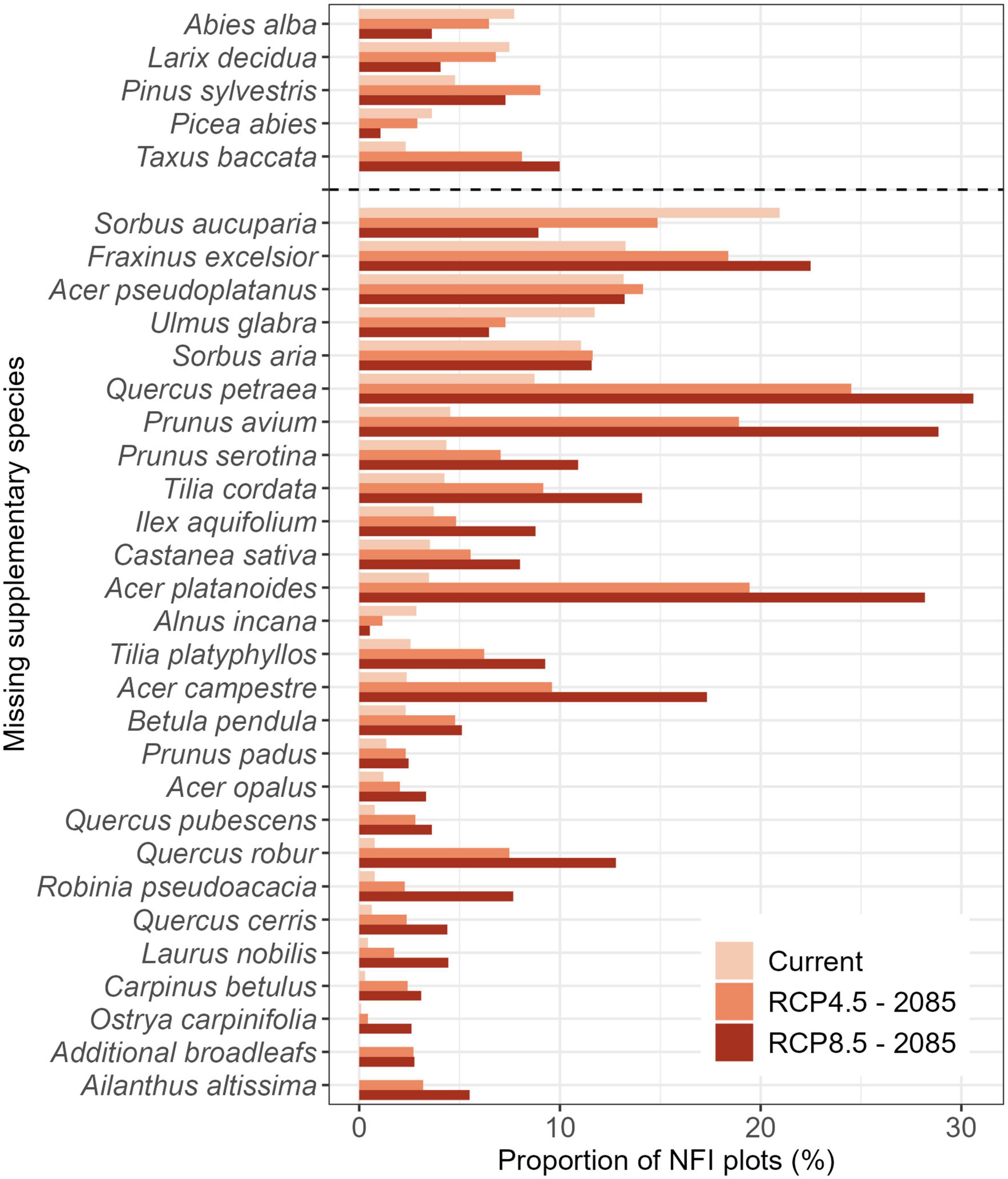
Figure 7. Proportion of National Forest Inventory (NFI) plots lacking a tree species that should be an important supplementary element of the current or future protective mountain forest stand based on the Natural forest Site Types (NST). Species are separated into conifers (top) and broadleaves (bottom). Only species missing from more than 2.5% of the NFI plots in protective forests are shown (for all species see Supplementary Figure 6).
Discussion
Based on the NFI data, tree species composition of almost 40% of protective mountain forests did not match tree species composition of the potential natural forest (NSTcurrent). As this mismatch is predicted to further increase with ongoing environmental change (NSTRCP4.5, RCP8.5), many protective mountain forests might pose a risk in regard to reduced stability and higher disturbance susceptibility both potentially undermining their protective function (Elkin et al., 2013; Albrich et al., 2018).
Current and future drivers of naturalness
The two main tree species negatively impacting naturalness due to their over-representation are Norway spruce and European larch, which relates to past management practices favoring these two species for diverging reasons. Norway spruce has been and still is the main timber species used in Switzerland (Bundesamt für Statistik, 2018; Cioldi et al., 2020). European larch, on the other hand, was for long favored by farmers to create more sunlight forests, i.e., pastured woodland, enabling more productive grazing (Meyer, 1951). As European larch is profiting from high disturbance frequencies (especially avalanches) and lower susceptibility to snow related damages and diseases (Meyer, 1955), it is abundant in abandoned pastured woodlands and in avalanche paths. All conifers, especially Norway spruce and European larch, are projected to have a diminished importance in future protective mountain forests, at the cost of increasingly climate-suitable European beech and other broadleaf species such as oaks and limes. The NSTs projected for the future also suggest an increasing importance of ash and elm. However, ash is heavily affected by ash dieback (Queloz et al., 2017; Enderle et al., 2019) and elm by Dutch elm disease (Karnosky, 1979; Nierhaus-Wunderwald and Engesser, 2003). These two invasive pathogens make it unlikely that ash and elm will increase in proportion unless resistant variants are found, planted, and promoted (Smalley and Guries, 1993; Klesse et al., 2021; Martín et al., 2021). Another important broadleaf species hindered by diseases is sweet chestnut (Meyer et al., 2015; Rigling and Prospero, 2018). While sweet chestnut is not expected to increase in overall dominance, the species remains locally important.
With ongoing climate warming, many mountain forest stands ought to transform from conifer to broadleaf dominated to reach equilibrium with environmental conditions (Albrich et al., 2020). The potential future tree species pool (NSTRCP4.5, NSTRCP8.5) also includes several neophytes [e.g., Pseudotsuga menziesii (Mirb.) Franco, Ailanthus altissima (Mill.) Swingle, Robinia pseudoacacia L.]. These species are climatically well-suited for the expected future conditions and potentially can contribute to the protective function of forests (Eilmann and Rigling, 2012; Lévesque et al., 2014; Knüsel et al., 2015, 2019). However, these neophytes might be very problematic regarding other ecosystem services such as biodiversity and might therefore not be planted or even actively removed, especially if they are on the blacklist for invasive species (BAFU, 2022). In addition, forests invaded by these exotic species might have a lower protective effect against the prevalent natural hazard (Knüsel et al., 2015; Moos et al., 2019).
Impacts of low naturalness on protective forests
Low naturalness of tree composition directly impacts the main ecosystem service of protective forests, as many criteria used to determine the protective capabilities of a forest against a certain natural hazard (e.g., avalanches, rockfall and landslides), are linked to species mixtures (Frehner et al., 2005). Our study was based on NFI plot data (i.e., maximum 0.25 ha), an area considerably smaller than recommended for the field evaluation (1–1.5 ha) of the protective effects of mountain forests according to NaiS. While this scale mismatch might explain some of the divergences in species composition, it is apparent that many mountain forests show reduced naturalness, especially if assumed dominant tree species of the potential natural forest are not even present in the NFI plots.
Low naturalness might not necessarily indicate insufficient protective function, e.g., if the number of stems and gap sizes are within the desired parameters to protect efficiently against rockfall. However, low naturalness has been shown to increase the susceptibility of forest stands to detrimental disturbances (Scherrer et al., 2023a) disrupting essential ecosystem services especially in conifer dominated stands (Thom and Seidl, 2016; Stritih et al., 2021; Scherrer et al., 2022). In the long-term it is desirable to transform the tree species composition of mountain forest stands to reach a (pseudo-)equilibrium with the environmental conditions. However, this might be challenging for several reasons, especially, if important tree species that should currently or in the future dominate or co-dominate are missing today. With accelerating climate warming the principle of natural regeneration alone is unlikely to be expedient to adapt/transform mountain forests if key species are missing from the seed pool even if favorable micro-sites are generated for the target species (Schönenberger, 2001). Even if those species reach climatically favorable micro-sites they might still fail to establish under current conditions due to biological factors such as competition of existing dominant tree species and browsing (Kupferschmid et al., 2019; Abegg et al., 2020; Scherrer et al., 2020). In fact, it is assumed that the regeneration of current and future key species such as silver fir or oak is mostly hampered due to a high browsing pressure, mainly by deer (Kupferschmid et al., 2015; Abegg et al., 2020). Given the slow growth rates of high elevation forests it is unlikely that species currently only present as single seedlings will rapidly, i.e., within the next decades, develop into stand dominating tree species. As a result of this seed source limitation the missing tree species are often planted to improve recruitment success (Wohlgemuth et al., 2017; Frehner and Plozza, 2018). However, planting of tree seedling considerably increases management requirements and associated costs as these seedlings often need artificial protection from browsing damage by deer.
Continued protective function in a changing environment
Ongoing climate change forces protective mountain forests to adapt (i.e., change their tree species composition) to stay in equilibrium with environmental conditions minimizing the risk of ecosystem failures (Albrich et al., 2018). However, several studies showed that (undisturbed) forests are highly inert to gradual change (Küchler et al., 2015; Scherrer et al., 2020). It means that despite recent climate warming, many undisturbed forests persist in terms of species composition (Küchler et al., 2015; Scherrer et al., 2017), due to asymmetric competition, seed load of the existing dominant climax species (Lenoir et al., 2010; Scherrer et al., 2021) and browsing pressure (Côté et al., 2004; Didion et al., 2009; Cailleret et al., 2014). The aforementioned likely increases the risk of stand level disturbances leading to sudden dieback of large proportions of the stand forming trees (Albrich et al., 2018; Scherrer et al., 2023a). After such a stand-replacing disturbance the tabula- rasa-situation allows for the establishment of adapted pioneer tree species and ultimately will develop toward a new climax forests (Scherrer et al., 2022). However, this succession processes take decades to centuries to reach a new climax state, especially in mountain forests (Schumacher and Bugmann, 2006). While large-scale disturbances and associated temporary loss of certain ecosystem functions might be unproblematic or even beneficial in nature reserves (e.g., Müller et al., 2008), the continued ecosystem service provisioning is the key target for protective mountain forests to reduce socio-economic risks (e.g., Losey and Wehrli, 2013; Teich et al., 2019).
Especially, the combination of the projected over-representation of Norway spruce with accelerated climate warming might prove a major risk factor (Scherrer et al., 2023a). Next to increasing weather extremes, increased temperatures will allow for multiple generations of bark beetles per year, even at high elevation sites, multiplying the pressure on susceptible Norway spruce stands (Stadelmann et al., 2014; Caduff et al., 2022). Once a stand is “destroyed” by a bark beetle attack or wind throw it takes decades before the protective function is restored by natural regeneration and succession dynamics (Schwarz, 2019; Caduff et al., 2022; Moos et al., 2022). The target is therefore a gradual adaptation of the tree species composition in accordance with the environmental change. This might be challenging if forest stands need to be transformed from conifer to broadleaf dominated and if important future tree species are currently absent. In cases where seed trees of important current/future species are missing despite already favorable climatic conditions supplementary planting is advisable to accelerate forests transformation and adaptation. In addition, the browsing pressure needs to be regulated to allow for establishment and natural regeneration of desired tree species to ensure a stable forest in the longer term.
In our view, the concept of naturalness is a useful tool for guiding forest management of protective mountain forests, ensuring continued forest stability (i.e., resilience and resistance). However, the concept has its limitation and numerous other factors must be considered when managing protective mountain forests e.g., the uncertainty of climate projections, potential spread and emergency of pathogens, society driven changes in priorities of ecosystem services.
Furthermore, it remains the question which natural hazards the forests will need to protect against in the future and if the protective effect of the forests will be still the same. While avalanches might decrease with climate warming, landslides and rockfall might increase (Bebi et al., 2016). Climate warming is shifting the suitability of conifer dominated forest to higher elevations, while the objects the forest should protect (i.e., roads or houses), stay in place. The different stand architecture of conifer and broadleaf dominated forests might therefore cause problems to sustainably provide the hitherto large breath of protective functions of conifer forests against natural hazards (e.g., Stokes et al., 2005; Wehrli et al., 2006). Transitioning from evergreen conifers to deciduous broadleaves might be problematic regarding avalanches but broadleaves protect equally or better against rockfall (Berger and Dorren, 2007) and landslides than conifers (Schwarz, 2019). Ongoing research can provide useful information to forest managers about the growth, regeneration, and protective potential of different tree species mixtures and provenances under the expected future conditions. This information should not only be based on model predictions but supported by experimental data from provenance research (e.g., Martínez-Sancho et al., 2021; Wang et al., 2022), climate manipulation experiments (e.g., Schönbeck et al., 2022) and long-terms observations (e.g., Frei et al., 2018; Bogdziewicz et al., 2020).
Data availability statement
The data and R-scripts used in this manuscript are stored in the EnviDat repository (https://www.doi.org/10.16904/envidat.400; Scherrer et al., 2023b). The raw data from the Swiss NFI can be provided free of charge within the scope of a contractual agreement (http://www.lfi.ch/dienstleist/daten-en.php).
Author contributions
DS: methodology, formal analysis, writing—original draft, and visualization. BA and MF: resources, writing—review, and editing. CF: data curation, writing—review, and editing. TW: conceptualization, writing—original draft, funding acquisition, and supervision. All authors contributed to the article and approved the submitted version.
Funding
Open access funding provided by Lib4RI—Library for the Research Institutes within the ETH Domain: Eawag, Empa, PSI & WSL and Swiss Federal Institute for Forest, Snow and Landscape Research (WSL).
Conflict of interest
The authors declare that the research was conducted in the absence of any commercial or financial relationships that could be construed as a potential conflict of interest.
Publisher’s note
All claims expressed in this article are solely those of the authors and do not necessarily represent those of their affiliated organizations, or those of the publisher, the editors and the reviewers. Any product that may be evaluated in this article, or claim that may be made by its manufacturer, is not guaranteed or endorsed by the publisher.
Supplementary material
The Supplementary Material for this article can be found online at: https://www.frontiersin.org/articles/10.3389/ffgc.2023.1191639/full#supplementary-material
Footnotes
References
Abegg, M., Huber, M., and Düggelin, C. (2020). “Gesundheit und Vitalität,” in Schweizerisches Landesforstinventar. Ergebnisse der Vierten Erhebung 2009-2017, eds U. B. Brändli, M. Abegg, and B. Allgaier Leuch (Birmensdorf: Eidgenössische Forschungsanstalt für Wald, Schnee und Landschaft WSL; Bern: Bundesamt für Umwelt BAFU).
Acharya, R. P., Maraseni, T., and Cockfield, G. (2019). Global trend of forest ecosystem services valuation–An analysis of publications. Ecosyst. Serv. 39:100979. doi: 10.1016/j.ecoser.2019.100979
Albrich, K., Rammer, W., and Seidl, R. (2020). Climate change causes critical transitions and irreversible alterations of mountain forests. Glob. Chang. Biol. 26, 4013–4027. doi: 10.1111/gcb.15118
Albrich, K., Rammer, W., Thom, D., and Seidl, R. (2018). Trade-offs between temporal stability and level of forest ecosystem services provisioning under climate change. Ecol. Appl. 28, 1884–1896. doi: 10.1002/eap.1785
ARGE Frehner, M., Dionea, S. A., and IWA - Wald und Landschaft AG (2020). NaiS-LFI – Zuordnung der LFI-Stichprobenpunkte zu Waldgesellschaften. Erläuternder Schlussbericht. Bern: Auftrag des Bundesamt für Umwelt BAFU.
BAFU (2022). Gebietsfremde Arten in der Schweiz. Übersicht über die Gebietsfremden Arten und ihre Auswirkungen. Bern: Umwelt-Wissen.
Bebi, P., Bugmann, H., Lüscher, P., Lange, B., and Brang, P. (2016). “Auswirkungen des Klimawandels auf Schutzwald und Naturgefahren,” in Wald im Klimawandel. Grundlagen für Adaptationsstrategien, eds A. R. Pluess, S. Augustin, and P. Brang (Bern: Bundesamt für Umwelt BAFU; Birmensdorf; Eidg. Forschungsanstalt WSL; Bern, Stuttgart, Wien: Haupt).
Berger, F., and Dorren, L. K. A. (2007). Principles of the tool Rockfor.net for quantifying the rockfall hazard below a protection forest. Schweiz. Z. fur Forstwes. 158, 157–165. doi: 10.3188/szf.2007.0157
Bogdziewicz, M., Kelly, D., Thomas, P. A., Lageard, J. G. A., and Hacket-Pain, A. (2020). Climate warming disrupts mast seeding and its fitness benefits in European beech. Nature Plants 6, 88–94. doi: 10.1038/s41477-020-0592-8
Bowditch, E., Santopuoli, G., Binder, F., del Río, M., La Porta, N., and Kluvankova, T. (2020). What is climate-smart forestry? a definition from a multinational collaborative process focused on mountain regions of Europe. Ecosyst. Serv. 43:101113. doi: 10.1016/j.ecoser.2020.101113
Brändli, U. B., Abegg, B., Allgaier-Leuch, B., Bischof, S., Cioldi, F., Düggelin, C., et al. (2020a). “Das Landesforstinventar,” in Schweizerisches Landesforstinventar Ergebnisse der Vierten Erhebung 2009–2017, eds U. B. Brändli, B. Abegg, and B. Allgaier-Leuch (Birmensdorf: Eidg. Forschungsanstalt für Wald, Schnee und Landschaft; Bern: Bundesamt für Umwelt).
Brändli, U. B., Abegg, M., and Düggelin, C. (2020b). “Biologische Vielfalt,” in Schweizerisches Landesforstinventar: Ergebnisse der Vierten Erhebung 2009–2017, eds U. B. Brändli, M. Abegg, and B. Allgaier Leuch (Birmensdorf: Eidgenössische Forschungsanstalt für Wald, Schnee und Landschaft WSL; Bern: Bundesamt für Umwelt, BAFU).
Brang, P., Spathelf, P., Larsen, J. B., Bauhus, J., Boncèìna, A., Drössler, L., et al. (2014). Suitability of close-to-nature silviculture for adapting temperate European forests to climate change. For. Int. J. For. Res. 87, 492–503. doi: 10.1093/forestry/cpu018
Breshears, D. D., López-Hoffman, L., and Graumlich, L. J. (2011). When ecosystem services crash: preparing for big, fast, patchy climate change. AMBIO 40, 256–263. doi: 10.1007/s13280-010-0106-4
Bundesamt für Statistik. (2018). Eidg. Holzverarbeitungserhebung 2017 - Rundholzeinschnitt, Schnitt- und Restholz in den Sägereien in m3 Nach Kantonen. Bern: Bundesamt für Statistik.
Bürgi, M. (2015). “Close-to-nature forestry,” in Europe’s Changing Woods and Forests: from Wildwood to Managed Landscapes, eds K. Kirby and C. Watkins (Wallingford: CABI).
Caduff, M. E., Brožová, N., Kupferschmid, A. D., Krumm, F., and Bebi, P. (2022). How large-scale bark beetle infestations influence the protective effects of forest stands against avalanches: a case study in the Swiss Alps. For. Ecol. Manage 514:120201. doi: 10.1016/j.foreco.2022.120201
Cailleret, M., Heurich, M., and Bugmann, H. (2014). Reduction in browsing intensity may not compensate climate change effects on tree species composition in the Bavarian Forest National Park. For. Ecol. Manage 328, 179–192.
CH2018 (2018). CH2018 - Climate Scenarios for Switzerland, Technical Report. Zürich: National Centre for Climate Services.
Cioldi, F., Brändli, U. B., Didion, M., Fischer, C., Ginzler, C., Herold, A., et al. (2020). “Waldresourcen,” in Schweizerisches Landesforstinventar. Ergebnisse der Vierten Erhebung 2009-2017, eds U. B. Brändli, M. Abegg, and B. Allgaier-Leuch (Birmensdorf: Eidgenössische Forschungsanstalt für Wald, Schnee und Landschat WSL; Bern: Bundesamt fur Umwelt BAFU).
Côté, S. D., Rooney, T. P., Tremblay, J.-P., Dussault, C., and Waller, D. M. (2004). Ecological impacts of deer overabundance. Annu. Rev. Ecol. Evol. Syst. 35, 113–147.
Daniel, C. J., Ter-Mikaelian, M. T., Wotton, B. M., Rayfield, B., and Fortin, M.-J. (2017). Incorporating uncertainty into forest management planning: timber harvest, wildfire and climate change in the boreal forest. For. Ecol. Manage 400, 542–554. doi: 10.1016/j.foreco.2017.06.039
Didion, M., Kupferschmid, A. D., and Bugmann, H. (2009). Long-term effects of ungulate browsing on forest composition and structure. For. Ecol. Manage 258, S44–S55. doi: 10.1007/s00442-019-04516-8
Dyderski, M. K., Paź, S., Frelich, L. E., and Jagodziński, A. M. (2018). How much does climate change threaten European forest tree species distributions? Glob. Chang. Biol. 24, 1150–1163. doi: 10.1111/gcb.13925
Eilmann, B., and Rigling, A. (2012). Tree-growth analyses to estimate tree species’ drought tolerance. Tree Physiol. 32, 178–187. doi: 10.1093/treephys/tps004
Elkin, C., Gutiérrez, A. G., Leuzinger, S., Manusch, C., Temperli, C., Rasche, L., et al. (2013). A 2° C warmer world is not safe for ecosystem services in the European Alps. Glob. Chang. Biol. 19, 1827–1840. doi: 10.1111/gcb.12156
Ellenberg, H., and Klötzli, F. (1972). Waldgesellschaften und Waldstandorte der Schweiz. Mitteilungen der Schweizerischen Anstalt für das forstliche Versuchswesen. Zürich: Beer.
Enderle, R., Stenlid, J., and Vasaitis, R. (2019). An overview of ash (Fraxinus spp.) and the ash dieback disease in Europe. CAB Rev. 14, 1–12. doi: 10.1079/PAVSNNR201914025
Fischer, C., and Traub, B. (2019). Swiss National Forest Inventory-Methods and Models of the Fourth Assessment. Berlin: Springer.
Frehner, M., Burnand, J., Carraro, G., Frey, H., and Lüscher, P. (2009). Nachhaltigkeit und Erfolgskontrolle im Schutzwald Wegleitung für Pflegemassnahmen in Wäldern mit Schutzfunktion Anhang 2A: Bestimmen des Standortstyps. Bern: Bundesamt für Umwelt, Wald und Landschaft.
Frehner, M., Huber, B., Gubelmann, P., Zürcher-Gasser, N., Zimmermann, N. E., Braun, S., et al. (2019). Schlussbericht des Projektes Adaptierte Ökogramme im Forschungsprogramm Wald und Klimawandel. Switzerland: ETH Zurich.
Frehner, M., and Plozza, L. (2018). Waldbrand Misox: Pflanzungen mit Berücksichtigung des Klimawandels. Bündner Wald 71, 20–25.
Frehner, M., Wasser, B., and Schwitter, R. (2005). Nachhaltigkeit und Erfolgskontrolle im Schutzwald. Wegleitung für Pflegemassnahmen in Wäldern mit Schutzfunktion. Bern: Bundesamt für Umwelt, Wald und Landschaft.
Frei, E. R., Streit, K., and Brang, P. (2018). Testpflanzungen zukunftsfähiger Baumarten: Auf dem Weg zu einem schweizweiten Netz. Schweiz. Z. Forst. 169, 347–350.
Frey, H., Frehner, M., Burnand, J., Carraro, G., and Rutishauser, U. (2021). Zur Entstehung der NaiS-Standortstypen. Schweiz. Z. Forst. 172, 216–225. doi: 10.3188/szf.2021.0146
Huber, B., Frehner, M., Zimmermann, N. E., Gubelmann, P., and Wüest, R. (2017). Vorarbeiten für Baumartenempfehlungen von Standortstypen, die in der Schweiz heute noch nicht vorkommen: Ein Bericht aus dem Projekt Adaptierte Ökogramme im Forschungsprogramm Wald und Klimawandel. Switzerland: ETH Zurich.
Karnosky, D. F. (1979). Dutch elm disease: a review of the history, environmental implications, control, and research needs. Environ. Conserv. 6, 311–322.
Klesse, S., Abegg, M., Hopf, S. E., Gossner, M. M., Rigling, A., and Queloz, V. (2021). Spread and severity of ash dieback in Switzerland–tree characteristics and landscape features explain varying mortality probability. Front. For. Glob. Change 4:645920. doi: 10.3389/ffgc.2021.645920
Knüsel, S., Conedera, M., Rigling, A., Fonti, P., and Wunder, J. (2015). A tree-ring perspective on the invasion of Ailanthus altissima in protection forests. For. Ecol. Manage 354, 334–343.
Knüsel, S., Conedera, M., Zweifel, R., Bugmann, H., Etzold, S., and Wunder, J. (2019). High growth potential of Ailanthus altissima in warm and dry weather conditions in novel forests of southern Switzerland. Trees 33, 395–409.
Koca, D., Smith, B., and Sykes, M. T. (2006). Modelling regional climate change effects on potential natural ecosystems in Sweden. Clim. Change 78, 381–406.
Küchler, M., Küchler, H., Bedolla, A., and Wohlgemuth, T. (2015). Response of Swiss forests to management and climate change in the last 60 years. Ann. For. Sci. 72, 311–320. doi: 10.1007/s13595-014-0409-x
Kupferschmid, A. D., Bütikofer, L., Hothorn, T., Schwyzer, A., and Brang, P. (2019). Quantifying the relative influence of terminal shoot browsing by ungulates on tree regeneration. For. Ecol. Manage. 446, 331–344. doi: 10.1016/j.foreco.2019.05.009
Kupferschmid, A. D., Wasem, U., and Bugmann, H. (2015). Browsing regime and growth response of Abies alba saplings planted along light gradients. Eur. J. For. Res. 134, 75–87.
Lanz, A., Fischer, C., and Abegg, M. (2019). Sampling Design and Estimation Procedures, Swiss National Forest Inventory–Methods and Models of the Fourth Assessment. Berlin: Springer.
Lenoir, J., Bertrand, R., Comte, L., Bourgeaud, L., Hattab, T., Murienne, J., et al. (2020). Species better track climate warming in the oceans than on land. Nat. Ecol. Evol. 4, 1044–1059.
Lenoir, J., Gegout, J. C., Dupouey, J. L., Bert, D., and Svenning, J. C. (2010). Forest plant community changes during 1989-2007 in response to climate warming in the Jura Mountains (France and Switzerland). J. Veg. Sci. 21, 949–964.
Lévesque, M., Rigling, A., Bugmann, H., Weber, P., and Brang, P. (2014). Growth response of five co-occurring conifers to drought across a wide climatic gradient in Central Europe. Agric. For. Meteorol. 197, 1–12. doi: 10.1016/j.agrformet.2014.06.001
Losey, S., and Wehrli, A. (2013). Schutzwald in der Schweiz. Vom Projekt SilvaProtect-CH zum harmonisierten Schutzwald. Bern: Bundesamt Umwelt.
Martí,n, J. A., Domínguez, J., Solla, A., Brasier, C. M., Webber, J. F., Santini, A., et al. (2021). Complexities underlying the breeding and deployment of Dutch elm disease resistant elms. New For. 1–36. doi: 10.1007/s11056-021-09865-y
Martínez-Sancho, E., Rellstab, C., Guillaume, F., Bigler, C., Fonti, P., Wohlgemuth, T., et al. (2021). Post-glacial re-colonization and natural selection have shaped growth responses of silver fir across Eur. Sci. Total Environ. 779:146393. doi: 10.1016/j.scitotenv.2021.146393
Mathys, A., Bottero, A., Stadelmann, G., Thürig, E., Ferretti, M., and Temperli, C. (2021). Presenting a climate-smart forestry evaluation framework based on national forest inventories. Ecol. Indic. 133:108459. doi: 10.1016/j.ecolind.2021.108459
Meyer, J. B., Gallien, L., and Prospero, S. (2015). Interaction between two invasive organisms on the European chestnut: does the chestnut blight fungus benefit from the presence of the gall wasp? FEMS Microbiol. Ecol. 91:fiv122. doi: 10.1093/femsec/fiv122
Meyer, K. A. (1951). “Frühere Verbreitung der Holzarten und einstige Waldgrenze im Kanton Wallis II. Mittelwallis,” in Mitteilungen der Schweizerischen Anstalt für das Forstliche Versuchswesen, ed. H. Burger (Zürich: Beer & CIE).
Meyer, K. A. (1955). “Frühere Verbreitung der Holzarten und einstige Waldgrenze im Kanton Wallis IV. Oberwallis,” in Mitteilungen der Schweizerischen Anstalt für das Forstliche Versuchswesen, ed. A. Kurth (Zürich: Beer & CIE).
Miura, S., Amacher, M., Hofer, T., San-Miguel-Ayanz, J., and Thackway, R. (2015). Protective functions and ecosystem services of global forests in the past quarter-century. For. Ecol. Manage 352, 35–46. doi: 10.1016/j.foreco.2015.03.039
Moos, C., May, D., Dorren, L., Temperli, C., and Schwarz, M. (2022). Quantifying the Temporal Dynamics of the Protective Effect of Forests Against Rockfall After Disturbances.
Moos, C., Toe, D., Bourrier, F., Knüsel, S., Stoffel, M., and Dorren, L. (2019). Assessing the effect of invasive tree species on rockfall risk – the case of Ailanthus altissima. Ecol. Eng. 131, 63–72. doi: 10.1016/j.ecoleng.2019.03.001
Müller, J., Bußler, H., Goßner, M., Rettelbach, T., and Duelli, P. (2008). The European spruce bark beetle Ips typographus in a national park: from pest to keystone species. Biodivers. Conserv. 17, 2979–3001. doi: 10.1007/s10531-008-9409-1
Nabuurs, G.-J., Delacote, P., Ellison, D., Hanewinkel, M., Hetemäki, L., and Lindner, M. (2017). By 2050 the mitigation effects of EU forests could nearly double through climate smart forestry. Forests 8:484. doi: 10.3390/f8120484
Nierhaus-Wunderwald, D., and Engesser, R. (2003). Ulmenwelke - Biologie, Vorbeugung und Gegenmassnahmen. Merkblatt für die Praxis 20, 6pp (Birmensdorf: Eidg. Forschungsanstalt WSL).
Ninan, K. N. (ed.) (2014). Valuing ecosystem services: Methodological issues and case studies. Cheltenham: Edward Elgar Publishing.
Ott, E., Frehner, M., Frey, H.-U., and Lüscher, P. (1997). Gebirgsnadelwälder: Ein Praxisorientierter Leitfaden für eine standortgerechte Waldbehandlung. Switzerland: Obwalden.
Pluess, A. R., Augustin, S., and Brang, P. (2016). Wald im Klimawandel: Grundlagen für Adaptationsstrategien. Switzerland: Haupt Verlag Bern.
Prentice, I. C., Cramer, W., Harrison, S. P., Leemans, R., Monserud, R. A., and Solomon, A. M. (1992). A global biome model based on plant physiology and dominance, soil properties and climate. J. Biogeogr. 19, 117–134.
Queloz, V., Hopf, S., Schoebel, C., Rigling, D., and Gross, A. (2017). “Ash dieback in Switzerland: history and scientific achievements,” in Dieback of European ash (Fraxinus spp.): Consequences and Guidelines for Sustainable Management, eds R. Vasaitis and R. Enderle (Brussels: European Cooperation in Science & Technology).
Rigling, D., and Prospero, S. (2018). Cryphonectria parasitica, the causal agent of chestnut blight: invasion history, population biology and disease control. Mol. Plant Pathol. 19, 7–20. doi: 10.1111/mpp.12542
Runting, R. K., Bryan, B. A., Dee, L. E., Maseyk, F. J. F., Mandle, L., Hamel, P., et al. (2017). Incorporating climate change into ecosystem service assessments and decisions: a review. Glob. Chang. Biol. 23, 28–41.
Santopuoli, G., Temperli, C., Alberdi, I., Barbeito, I., Bosela, M., Bottero, A., et al. (2021). Pan-European sustainable forest management indicators for assessing climate-smart forestry in Europe. Can. J. For. Res. 51, 1741–1750.
Scherrer, D., Ascoli, D., Conedera, M., Fischer, C., Maringer, J., Moser, B., et al. (2022). Canopy disturbances catalyse tree species shifts in swiss forests. Ecosystems 25, 199–214.
Scherrer, D., Baltensweiler, A., Bürgi, M., Fischer, C., Stadelmann, G., and Wohlgemuth, T. (2023a). Low naturalness of Swiss broadleaf forests increases their susceptibility to disturbances. For. Ecol. Manage. 532:120827.
Scherrer, D., Fischer, C., and Frehner, M. (2023b). Naturalness of Tree Species Composition in Protective Forests. EnviDat. doi: 10.16904/envidat.400
Scherrer, D., Hiltebrand, F., Dengler, J., and Wohlgemuth, T. (2021). Mind the gaps: comparison of representative vs opportunistic assessment of tree regeneration in Central European beech forests. For. Ecol. Manage. 491:119179.
Scherrer, D., Massy, S., Meier, S., Vittoz, P., and Guisan, A. (2017). Assessing and predicting shifts in mountain forest composition across 25 years of climate change. Divers. Distrib. 23, 517–528.
Scherrer, D., Vitasse, Y., Guisan, A., Wohlgemuth, T., and Lischke, H. (2020). Competition and demography rather than dispersal limitation slow down upward shifts of trees’ upper elevation limits in the Alps. J. Ecol. 108, 2416–2430.
Schönbeck, L., Grossiord, C., Gessler, A., Gisler, J., Meusburger, K., D’Odorico, P., et al. (2022). Photosynthetic acclimation and sensitivity to short-and long-term environmental changes in a drought-prone forest. J. Exp. Bot. 73, 2576–2588.
Schönenberger, W. (2001). Trends in mountain forest management in Switzerland. Schweiz. Z. Forst. 152, 152–156.
Schumacher, S., and Bugmann, H. (2006). The relative importance of climatic effects, wildfires and management for future forest landscape dynamics in the Swiss Alps. Glob. Change Biol. 12, 1435–1450.
Schütt, P., Weisgerber, H., Schuck, H. J., Lang, U. M., Roloff, A., Stimm, B., et al. (2006). Enzyklopädie der Holzgewächse. Handbuch und Atlas der Dendrologie. Ecomed Medizin. Heidelberg: Verlagsgruppe Hüthig Jehle Rehm GmbH.
Schwarz, M. (2019). Wurzelverstärkung und Hangstabilitätsberechnungen: ein Überblick. Schweiz Z. Forst. 170, 292–302.
Seidl, R., Thom, D., Kautz, M., Martin-Benito, D., Peltoniemi, M., Vacchiano, G., et al. (2017). Forest disturbances under climate change. Nat. Clim. Change 7, 395–402.
Smalley, E., and Guries, R. (1993). Breeding elms for resistance to Dutch elm disease. Annu. Rev. Phytopathol. 31, 325–354.
Spathelf, P., Bolte, A., and van der Maaten, E. (2015). Is close-to-nature silviculture (CNS) an adequate concept to adapt forests to climate change. Landbauforschung 65, 161–170.
Stadelmann, G., Bugmann, H., Wermelinger, B., and Bigler, C. (2014). Spatial interactions between storm damage and subsequent infestations by the European spruce bark beetle. For. Ecol. Manage 318, 167–174.
Stokes, A., Salin, F., Kokutse, A. D., Berthier, S., Jeannin, H., Mochan, S., et al. (2005). Mechanical resistance of different tree species to rockfall in the French Alps. Plant and Soil 278, 107–117.
Stritih, A., Bebi, P., Rossi, C., and Grêt-Regamey, A. (2021). Addressing disturbance risk to mountain forest ecosystem services. J. Environ. Manage. 296:113188.
Teich, M., and Bebi, P. (2009). Evaluating the benefit of avalanche protection forest with GIS-based risk analyses—a case study in Switzerland. For. Ecol. Manage 257, 1910–1919.
Teich, M., Giunta, A. D., Hagenmuller, P., Bebi, P., Schneebeli, M., and Jenkins, M. J. (2019). Effects of bark beetle attacks on forest snowpack and avalanche formation–implications for protection forest management. For. Ecol. Manage 438, 186–203.
Temperli, C., Blattert, C., Stadelmann, G., Brändli, U.-B., and Thürig, E. (2020). Trade-offs between ecosystem service provision and the predisposition to disturbances: a NFI-based scenario analysis. For. Ecosyst. 7:27.
Thom, D., Rammer, W., and Seidl, R. (2017). Disturbances catalyze the adaptation of forest ecosystems to changing climate conditions. Glob. Change Biol. 23, 269–282.
Thom, D., and Seidl, R. (2016). Natural disturbance impacts on ecosystem services and biodiversity in temperate and boreal forests. Biol. Rev. 91, 760–781.
Verkerk, P., Costanza, R., Hetemäki, L., Kubiszewski, I., Leskinen, P., Nabuurs, G., et al. (2020). Climate-smart forestry: the missing link. For. Policy Econ. 115:102164.
Wang, H., Lin, S., Dai, J., and Ge, Q. (2022). Modeling the effect of adaptation to future climate change on spring phenological trend of European beech (Fagus sylvatica L.). Sci. Total Environ. 846, 157540.
Wehrli, A., Dorren, L. K., Berger, F., Zingg, A., Schönenberger, W., and Brang, P. (2006). Modelling long-term effects of forest dynamics on the protective effect against rockfall. For. Snow Landsc. Res. 80, 57–76.
Wohlgemuth, T., Schwitter, R., Bebi, P., Sutter, F., and Brang, P. (2017). Post-windthrow management in protection forests of the Swiss Alps. Eur. J. For. Res. 136, 1029–1040.
Zimmermann, N. E., Normand, S., and Psomas, A. (2014). PorTree Final Report: a Project Funded by the BAFU-WSL Program on “Forests and Climate Change. Switzerland: ETH Zurich.
Keywords: bark beetle, hemeroby, Norway spruce, Switzerland, climate change, ecosystem services, Forest Inventory data
Citation: Scherrer D, Allgaier Leuch B, Fischer C, Frehner M and Wohlgemuth T (2023) Maintaining the protective function of mountain forests under climate change by the concept of naturalness in tree species composition. Front. For. Glob. Change 6:1191639. doi: 10.3389/ffgc.2023.1191639
Received: 22 March 2023; Accepted: 16 May 2023;
Published: 15 June 2023.
Edited by:
Christine Moos, Bern University of Applied Sciences, SwitzerlandReviewed by:
Olivier Bouriaud, Ştefan Cel Mare University of Suceava, RomaniaLuigi Portoghesi, University of Tuscia, Italy
Copyright © 2023 Scherrer, Allgaier Leuch, Fischer, Frehner and Wohlgemuth. This is an open-access article distributed under the terms of the Creative Commons Attribution License (CC BY). The use, distribution or reproduction in other forums is permitted, provided the original author(s) and the copyright owner(s) are credited and that the original publication in this journal is cited, in accordance with accepted academic practice. No use, distribution or reproduction is permitted which does not comply with these terms.
*Correspondence: Daniel Scherrer, daniel.scherrer@wsl.ch