From every day air travel to the awe-inspiring launch of space shuttles and satellites, we know that science is more than capable of taking us to the skies. But there is another, equally important field of research that flies under the radar, taking the form of Drosophila—the simple yet elegant fruit fly.
Hello, Drosophila
Meet the fruit fly, known among its closest friends as Drosophila melanogaster. You may have already encountered it during summer, buzzing around fruit bowls. Although unwanted guests at home, fruit flies are incredibly important allies in science research. They serve as one of the most widely used model organisms in labs all over the world.
At the time of writing, there have been six Nobel Prizes in Physiology in Medicine awarded to works where Drosophila was used (1933, 1946, 1995, 2004, 2011, 2017). Thousands of papers in scientific journals involve their use, from fields as varied as neuroscience, genetics, cancer research nutrition, sleep, development and evolution. A crew of fruit flies even became astronauts in 2015, sent to the International Space Station to study the effects of microgravity on organisms.
Model Organisms in Research
To understand their importance, we must first define what a ‘model organism’ is. Model organisms are non-human species used in research to understand biological systems. Theories and data gathered from these organisms should ideally be applicable to other organisms, especially to more complex ones such as humans1.
As such, model organisms are selected based on their suitability for experimentation and their degree of similarity to more complex animals. You might be wondering, how can the tiny fruit fly be compared to a rat, let alone the human body? Well, it can’t directly, but it does a good job representing biological phenomena that are similar in humans (for example, in early development).
In fact, hundreds of model organisms are currently being studied, each with its own niche. They are chosen based on their similarity to a certain process, suitability for genetic manipulation, cost and availability, etc. Some of the most used model organisms in research are Saccharomyces cerevisiae (yeast), Caenorhabditis elegans (nematode worm), Arabidopsis thaliana (plant), Danio rerio (zebrafish), Mus musculus (mouse), and of course, Drosophila melanogaster (fruit fly)2.
Early Drosophila melanogaster Research
The journey of D. melanogaster as a model organism began in 1901 at Harvard University, where American scientists Charles W. Woodworth and William E. Castle started breeding the little creatures in their labs. They were the first to suggest that fruit flies could be used to study genes, specifically how characteristics were passed from parent to offspring.
However, it was fellow scientist Thomas H. Morgan who turned fruit flies into research celebrities. With the help of his little assistants, Morgan discovered the role of chromosomes in heredity and evolution3. In 1909, he started breeding Drosophila and systematically screened them for mutations, producing experimental data that supported the theory of heredity and evolution.
One year later, Morgan and his team isolated a strange Drosophila mutant that had very different characteristics to its parents: it had white eyes instead of the usual red. Morgan concluded that this was caused by a silent or ‘recessive’ gene, with further cross-breeding showing that the gene was located on a sex-linked chromosome.

For the discovery of sex-linked recessive traits, Morgan was awarded the Nobel Prize in Physiology or Medicine in 1933. From that point on, many scientists started to direct research efforts toward the study of sex determination and inheritance using Drosophila as a model organism, ushering in a new era of genetics and evolution.
What Makes Fruit Flies Great Study Buddies?
Fruit flies are perfectly suited to be used as model organisms in a laboratory setting since they:
- Are easy to manipulate, and given their tiny size of around 3 mm in length and 2 mm in width, it is possible to keep large fly populations in a tiny space. Thousands of eggs, embryos, larvae, or adults can be collected and studied in a short time frame!
- Have a short life cycle. Each fruit fly lives for only about 10 days at 25°C, making it possible to perform studies across several generations in just a few weeks.
- Are cheap to grow and maintain. They are usually grown in little plastic bottles containing cornmeal and agar as food.
- Have had their entire genome completely sequenced and published since 2000.

Advancing Science and Medicine with Drosophila
Ok, so fruit flies are great news for scientists in the lab. But as a member of the public, you may be wondering, why should anyone else care about it? How can humans benefit from the study of this model organism?
Genetic Diseases
One of their main uses in research is to better understand and hopefully treat genetic diseases in humans. Around 75% of genetically-linked human diseases have counterparts present in D. melanogaster4. Despite the huge difference in size and appearance, an astonishing 60% of genes are conserved between fruit flies and humans5!
Among these shared genes are those involved in crucial early-stage processes, ‘telling’ cells how to divide, develop and function. Mutations in these genes can lead to diseases and malformations such as polydactyly—the formation of extra fingers and toes. By studying embryonic development, we can understand how our body structure is planned and where things can go wrong6.

Circadian Rhythm
Multiple life forms on earth have adapted their biology to the rotation of our planet, an example being the sleep-wake cycle in humans. Collectively, the biological changes that occur based on the time of day are known as the circadian rhythm. In 1984, a group of scientists discovered the gene responsible for producing a protein that properly regulates the sleep-wake cycle7,8. In 2017, three of them were awarded the Nobel prize in Physiology and Medicine, for their studies of circadian rhythm in fruit flies.
This sleep/wake protein, called PER, accumulates during the night and degrades during the day, forming a self-regulating clock. In Drosophila cells, PER levels oscillate over a 24-hour cycle in sync with its circadian rhythm. It was shown to regulate several activities such as movement, sensitivity to light, temperature changes and mating patterns, among others9,10.
Since this discovery, circadian biology has become an important field of research, with circadian processes having implications for our health11. The studies of how these processes are regulated in the fruit fly are also applicable to other animals, including humans12.

Immune System Response
In 1996, French biologist Jules Hoffmann and his group were studying Drosophila flies that carried a mutation in their developmental genes. They exposed the fruit flies to a certain fungus and realized that the mutant flies were more susceptible to infection than normal ones. They were unable to mount a proper immune response against the fungal attack.
Hoffmann made the discovery that certain developmental genes are also involved in pathogen detection and immune system response13. Meanwhile, in the U.S., geneticist Bruce Beutler identified the receptor in humans responsible for septic shock, a condition where the immune system is overwhelmed by infection14. The proteins that formed this receptor turned out to be similar to the developmental proteins in fruit flies! For these discoveries, Jules Hoffmann and Bruce Beutler shared the 2011 Nobel Prize in Physiology or Medicine.
Fruit Flies in Space
In the microgravity environment of space, cell behavior changes due to changes in how fluids move around in cells. This affects their size and shape, as well as their signaling and metabolism and division processes. In astronauts, these changes are known to cause bone and muscle loss, the latter posing a risk to normal heart function.

The International Space Station carries several model organisms on board to study the effects of microgravity. In particular, fruit flies are used to see how microgravity affects gene expression, along with how cellular and genetic mechanisms can cause heart problems during spaceflight. Scientists have even hatched fruit flies in space, comparing them with flies grown on Earth. Studying them in space could be useful to explore the effects of future long-duration spaceflights on human beings!
The Small but Mighty Fruit Fly
Highlighted above are just a small number of studies involving Drosophila melanogaster. Fruit flies are ideal model organisms in many areas, helping scientists understand the genetics of development and disease in humans. Next time you see one buzzing around the kitchen, you might think twice before squashing the mighty 6-time Nobel prize recipient!
This article was written by Julian Gurgo via Write For Us.
Reference
- What makes a model organism?, Sabina Leonelli and Rachel A. Ankeny, Endeavour, V.37, Issue 4, December 2013, Pages 209-212.
- Drosophila melanogaster as an experimental organism. GM Rubin, Science, 10 Jun 1988. Vol. 240, Issue 4858, pp. 1453-1459.
- Thomas Hunt Morgan at the Marine Biological Laboratory: Naturalist and Experimentalist. Diana E. Kenney and Gary G. Borisy. Genetics March 1, 2009 vol. 181 no. 3 841-846
- Homophila: human disease gene cognates in Drosophila. Samson Chien, Lawrence T. Reiter, Ethan Bier and Michael Gribskov. Nucleic Acids Research, 2002, Vol. 30, No. 1
- A Systematic Analysis of Human Disease-Associated Gene Sequences In Drosophila melanogaster. Lawrence T. Reiter, Lorraine Potocki, Sam Chien, Michael Gribskov, and Ethan Bier. Genome Res. 2001. 11: 1114-1125, Cold Spring Harbor Laboratory Press.
- The regulation of Hox gene expression during animal development. Moisés Mallo, Claudio R. Alonso. Development 2013 140: 3951-3963
- Zehring, W.A., Wheeler, D.A., Reddy, P., Konopka, R.J., Kyriacou, C.P., Rosbash, M., and Hall, J.C. (1984). P-element transformation with period locus DNA restores rhythmicity to mutant, arrhythmic Drosophila melanogaster. Cell 39, 369–376.
- Bargiello, T.A., Jackson, F.R., and Young, M.W. (1984). Restoration of circadian behavioural rhythms by gene transfer in Drosophila. Nature 312, 752–754.
- Circadian rhythms of female mating activity governed by clock genes in Drosophila. Takaomi Sakai, Norio Ishida. Proc Natl Acad Sci U S A. 2001 Jul 31; 98(16): 9221–9225.
- Temporal phosphorylation of the Drosophila period protein. I Edery, L J Zwiebel, M E Dembinska, and M Rosbash. PNAS March 15, 1994 91 (6) 2260-2264
- Exposure to light at night, nocturnal urinary melatonin excretion, and obesity/dyslipidemia in the elderly: a cross-sectional analysis of the HEIJO-KYO study. Obayashi K, Saeki K, Iwamoto J, Okamoto N, Tomioka K, Nezu S, Ikada Y, Kurumatani N. J Clin Endocrinol Metab. 2013;98:337–44.
- The genetics of mammalian circadian order and disorder: implications for physiology and disease. Takahashi JS, Hong HK, Ko CH, McDearmon EL. Nat Rev Genet. 2008;9:764–75.
- Lemaitre B, Nicolas E, Michaut L, Reichhart JM, Hoffmann JA. The dorsoventral regulatory gene cassette spätzle/Toll/cactus controls the potent antifungal response in drosophila adults. Cell 1996;86:973-983.
- Poltorak A, He X, Smirnova I, Liu MY, Van Huffel C, Du X, Birdwell D, Alejos E, Silva M, Galanos C, Freudenberg M, Ricciardi-Castagnoli P, Layton B, Beutler B. Defective LPS signaling in C3H/HeJ and C57BL/10ScCr mice: Mutations in Tlr4 gene. Science 1998;282:2085-2088.
About the Author
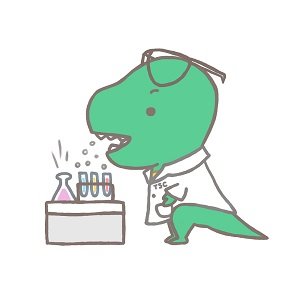
This article was written by a contributor. For a full list of guest writers, click here.